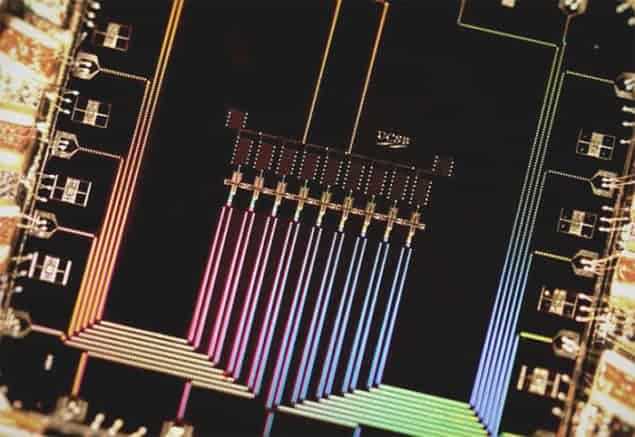
Bringing together the best of two types of quantum computer for the first time, researchers at Google have created a prototype that combines the architecture of both a universal quantum computer and an analogue quantum computer. By digitizing the traditionally analogue computations that can be done with an adiabatic quantum computer, the team’s system is one step closer to a universal quantum computer that could solve any computational problem. This is particularly relevant to some of the more complex and practical applications that scientists hope future quantum computers can tackle, including synthesizing new pharmaceutical drugs or deciphering long-term weather patterns.
A universal quantum computer is one that, in theory, can perform any computation exponentially faster than a classical computer. The race towards building a truly universal quantum computer currently involves a number of experimental groups and companies the world over – including Google, IBM and D-Wave – each of whom turn to different methods and technologies to achieve the same goal.
Slow build
Until now, both D-Wave and Google have focussed on “quantum annealing” – another method of quantum computing that is not universal but instead can be used to build an “adiabatic” quantum computer that can solve a specific problem which involves finding the global minimum value of a highly complex function. Annealing relies on “quantum tunnelling” and lets an initially simple system evolve very slowly towards the desired result. This involves encoding a problem into the states of some quantum bits (qubits) that have specifically assigned interactions. These interactions are traditionally classical, in that they are either on or off. The qubits are then put in a superposition of states and the system gradually evolves – ensuring that all the qubits always remain in the lowest energy “valley” or ground state – until the global minimum of the function is found.
While this method works fairly well for some problems, it is not the one-size-fits-all quantum computation technique that many scientists hope to create. Adiabatic quantum computing suffers from errors and noise because the process does not allow for error correction to take place during a computation. This becomes a major problem when the system is scaled up and errors accumulate. In contrast, a key component of many of the universal quantum computing architectures being developed is that their digital logic gates can be made fault-tolerant and error correction can take place while a calculation is being processed.
Digital age
Another problem with adiabatic quantum computing is the classical nature of the interactions – which puts a low limit on the number of other qubits that a qubit can interact with. For a quick computation, you would ideally want multiple interactions simultaneously taking place between all of the qubits. But in a complex computation, it would be nearly impossible to accurately keep track of these interactions. However, reducing the connectivity has a major impact on the system’s computational abilities.
To get around this dilemma, John Martinis, Rami Barends and colleagues at Google’s research laboratories in Santa Barbara, California, together with physicists at the University of California, Santa Barbara and the University of the Basque Country in Bilbao, Spain, have now added a digital component to their previously analogue device. By digitizing an adiabatic quantum computation, the team has a greater degree of control over the interactions between qubits, and they can also correct for errors while a computation is executed.
In the current work, the Google researchers have adapted their previously built superconducting nine-qubit chip, where interactions are controlled by connected logic gates that encode a problem. The team simulated a row of spin-coupled magnetic atoms in a chain. The atoms can have an aligned (ferromagnetic) or anti-aligned (anti-ferromagnetic) orientation – something that is a well-known problem in magnetochemistry. The researchers address each qubit individually via current pulses that tune into the inherent resonant frequency of their qubits, which have variable frequencies between 4 GHz and 5.5 GHz.
“In our architecture we can steer this frequency, much like you would tune a radio to a broadcast,” says Barends. He explains that they can tune the frequency of one qubit to that of another. “By moving qubit frequencies to or away from each other, interactions can be turned on or off. The exchange of quantum information resembles a relay race, where the baton can be handed down when the runners meet,” he adds. The team can even tune a qubit so that it is simultaneously in a superposition of being aligned and anti-aligned.
Scaling up
While this problem is one that a classical computer can easily crack today, the Google team’s digital approach can work on any type of interaction and is not limited by the connectivity between qubits. Barends tells physicsworld.com that “as a demonstration, we have implemented non-stoquastic interactions, something that is not possible with present-day analogue systems. This is important because problems that involve interacting electrons, like in quantum chemistry, are non-stoquastic.”
Also, digital adiabatic quantum computing is fully compatible with known quantum error-correction techniques. In an analogue system, each added qubit brings with it more noise and errors. “Arguably, this makes it difficult to get the most interesting applications, which require many degrees of freedom, to work on an analogue system. But with error correction, a digital implementation can, in principle, be scaled to an arbitrarily large number of qubits,” he explains. Currently though, there is no large-scale quantum error-corrected hardware, but it is something that many labs the world over are working towards.
The research is published in Nature.