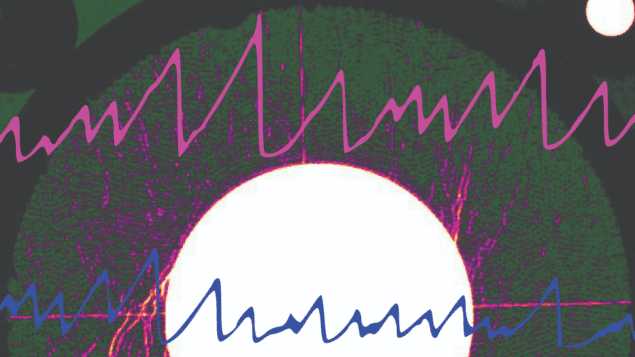
A new experiment has revealed how sheared granular materials emit sound waves that evolve in characteristic patterns as grains suddenly slip and rearrange themselves. The research, carried out by Ted Brzinski and Karen Daniels at North Carolina State University, could improve our ability to forecast natural disasters by monitoring the sounds emitted by granular materials in nature.
When granular materials experience shear forces – such as when tectonic plates rub against each other, or as the weight of snow on a steep slope acts against friction – the microscopically-vibrating grains will initially stick to the interface as stress builds in the material. When the stress becomes too high for the overall system to cope, many grains will slip at once; suddenly rearranging themselves into different patterns. During this stick-slip transition, grains develop low-frequency vibrational modes as stress is suddenly dissipated. The presence of these modes can be detected in the form of sound waves that are emitted at the material interface.
In a recent experiment, Daniels studied this effect by firing sound waves into granular materials and measuring how they changed as the sound had passed through. The study successfully documented how acoustic waves evolve in a characteristic pattern shortly before grains underwent stick-slip transitions. However, Daniels realized that manipulating the material directly made the technique somewhat invasive.
Rotating wall
In this latest study, she and Brzinski devised a way to observe the signals passively. To do this, the researchers created an annular chamber, with an inner wall that rotated once per hour, and a static outer wall. The space in between was filled with a single layer of 8000 small plastic disks, packed together as closely as possible to replicate a granular material. The disks resisted the rotation of the inner wall, which generated shear forces in the overall system. When stick-slip transitions eventually occurred, the disks rearranged themselves rapidly in about 0.5 s in a process that repeated roughly once every minute. The sounds produced by the events were then picked up by sensors embedded in the outer wall.

Graphene bubbles measure shear forces
As stress built up in the system, the sensor data revealed that individual disks vibrated in a narrow range of modes. This resulted in the generation of a spectrum of sound waves with similar frequencies, which did not evolve significantly over time. However, in the moments shortly before each slip, the frequency distribution of the disks’ vibrational modes began to broaden, while the average frequency increased gradually. After each slip, this average frequency dropped rapidly, and the distribution narrowed once again.
The researchers believe that the evolution they observed in the frequency distribution is characteristic enough to be useful for predicting slips in natural materials. Using sensors to measure changes in the sounds emitted at sites of potential avalanches, landslides or volcanic eruptions, it could become easier to predict when natural disasters are more likely to occur. Systems for predicting earthquakes would be a particularly useful application, although the researchers realise that they could still be a long way off. In the future, Brzinski and Daniels aim to collaborate with seismologists, which could allow them to develop some of the most sophisticated detection technology yet produced.
The research is described in Physical Review Letters.