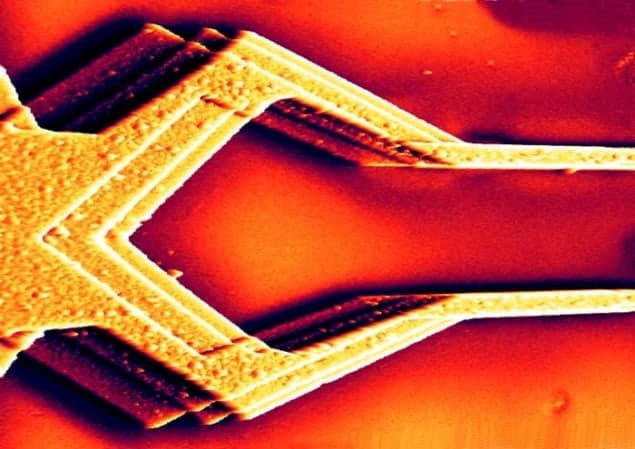
The thermal Josephson effect, which occurs when heat is transported across a gap between two superconductors, has been measured in the lab for the first time. The experiment was done by two physicists in Italy and confirms a theoretical prediction made in 1965. As well as confirming the bizarre prediction that some heat flows from the cold side of the junction to the hot side, the breakthrough could further the development of thermal circuits that use heat in much the same way as charge is used in electronic devices.
The conventional Josephson effect was predicted in 1962 by the British physicist Brian Josephson and was observed in the lab less than a year later. The effect occurs in a Josephson junction, which is created when two superconductors are separated by a thin layer of non-superconducting material or free space. Josephson showed that Cooper pairs – paired electrons that experience no electrical resistance within superconductors – can tunnel across the junction. All of the Cooper pairs are in the same quantum state and are thus represented by the same wavefunction. The tunnelling current is a sinusoidal function of the phase difference of the wavefunction from one side of the gap to the other. As a result, a current of Cooper pairs will flow across the gap even if there is no applied voltage.
If a voltage is applied across the junction, then things get more complicated and three different processes affect how current flows across the junction. The first, predominant term is the Josephson supercurrent, which would be present even without the voltage. The second contribution is from Cooper pairs that break apart into their constituent normal electrons, which can then tunnel through the potential barrier under the influence of the applied voltage. Last, there is an “interference current” that arises from the interaction between the first two processes.
Thermal bias
In 1965 Kazumi Maki and Allan Griffin of the University of California, San Diego calculated what would happen if a Josephson junction were given a thermal bias – that is, one side being slightly hotter than the other – rather than the usual voltage bias.
Ordinary metals can transport heat by energetic electrons moving from hot areas to colder regions – where they heat the surroundings by scattering from atoms and creating lattice vibrations. This does not apply to superconductors, however, because Cooper pairs move without scattering. In a thermally biased Josephson junction, therefore, the supercurrent does not contribute to heat flow. However, both electron tunnelling and the interference current can be involved in heat transfer across a junction.
Heat flow from electron tunnelling is a straightforward process that will always move heat from the hotter to the colder side of the junction. The curious part of Maki and Griffin’s prediction, however, is that the interference current can sometimes carry heat from the cold to the hot side. This is because, like the supercurrent, it depends on the superconductor wavefunction.
Tough to test
Testing this prediction has proved difficult, according to Francesco Giazotto and María José Martínez-Pérez of the NEST Institute of Nanoscience and Scuola Normale Superiore in Pisa – who are the first to do so. This is because, unlike electric currents, heat currents cannot be measured directly. “There is no analogue of an ammeter for a heat current so you are measuring an observable that is related only to the heat current like temperature,” explains Giazotto. He also says that it is much more difficult to keep track of heat flow than of charge.
Giazotto and Martínez-Pérez made their measurements on a superconducting quantum interference device (SQUID) – a loop of superconductor broken by two Josephson junctions. One half of the loop is kept at a slightly warmer temperature, causing normal electrons to carry heat across the two junctions to the cooler side as predicted.
The experiment involves altering the amount of magnetic flux that passes through the SQUID, which in turn affects the nature of the wavefuntion at a Josephson junction. By showing that the heat flow modulates between maximum and minimum values as the magnetic flux is changed, Giazotto and Martínez-Pérez have confirmed that the interference current can actually transfer heat from cold to hot. However, the overall heat flow does not reverse because the flow is dominated by normal electrons tunnelling through the junctions.
“Very clean result”
Raymond Simmonds of the National Institute for Standards and Technology in Boulder, Colorado, is impressed by the experimental challenges the researchers have overcome. “It’s really surprising that they got to measure a very clean result,” he says. “They had to do some pretty good engineering to make their heaters and their thermometry well characterized, all on a very small device, and to engineer all their contacts to ensure the system isn’t shorted out by the substrate.”
Giazotto and his colleagues are now look at possible practical applications of the result could be. He speculates about the possibility of “a sort of coherent caloritronic circuitry – the analogy of electronics but with heat”. He suggests it might be possible, for example, to produce heat transistors or heat rectifiers or even to produce devices without an electronic analogue.
The measurements are described in Nature.