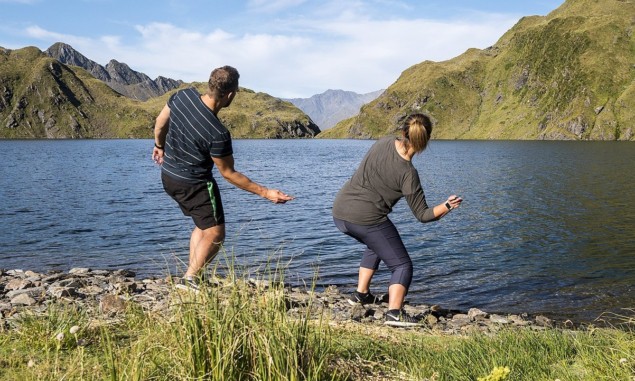
The physics that allows spinning stones to skip across the surface of water has been thoroughly analysed by Jie Tang at Southwest Jiaotong University in China and colleagues. The team used theoretical models and simple experiments to identify three key factors underlying the process and their findings could lead to important insights into the dynamics of aircraft and spacecraft that land on water.
Skipping a flat stone across water is one of the simple pleasures of life. With practice, imparting the right combination of throwing angle, speed, and spin will allow the stone to bounce several times before sinking. The physics involved in this process is also highly relevant in ensuring safe water landings for aircraft and re-entering spacecraft, which collide with water surfaces at high speeds.
In their study, Tang’s team constructed a mathematical model of stone skipping, which incorporated two key effects. The first of these was the Magnus effect, whereby the trajectory of a rotating object in a fluid is deflected – an effect that footballers use to send a ball on a curved trajectory. Secondly, the gyro effect involves the tendency of a spinning object to maintain a steady axis of rotation and travel in a straight line.
Navigation module
To verify their model’s predictions, the team did a simple experiment involving a spinning aluminium disk, fitted with a navigation module to measure its spin and trajectory during flight. Their setup enabled a tight control over the disk’s speed, rate of spin, and angle of approach to the water’s surface. Through a series of experiments, the team then measured how variations in each of these values affected the disk’s skipping dynamics.
From the results of their analysis, the researchers identified three key factors underlying these dynamics. The first factor is related to the upward acceleration of the disk – determined by its velocity and angle of approach to the water. If this value is over 4 g (four times the gravitational acceleration) the disk will skip. Yet at 3.8 g, the disk will instead “surf”, skimming along the water’s surface at an oscillating angle, but not bouncing.
The second factor is related to how the gyro effect can guarantee the stability of the disk’s angle of approach to the water, creating more favourable conditions for continuous bouncing. The third factor is that the direction of the disk’s trajectory reflects a combination of gyro and Magnus effects. For spin rates lower than 18 rotations per second, the Magnus effect will dominate, and the disk will veer off to the left or right, depending on its direction of spin. Yet above this rate, the gyro effect will dominate, and the disk will continue in a straight path.
If applied to the more complex shapes of air and spacecraft, Tang’s team hope that their results could improve our understanding of how flying vehicles behave when impacting water upon landing. This could soon enable engineers to design better vehicles and flight paths: ensuring both minimal damage to valuable equipment, and better safety for passengers.
The research is described in Physics of Fluids.