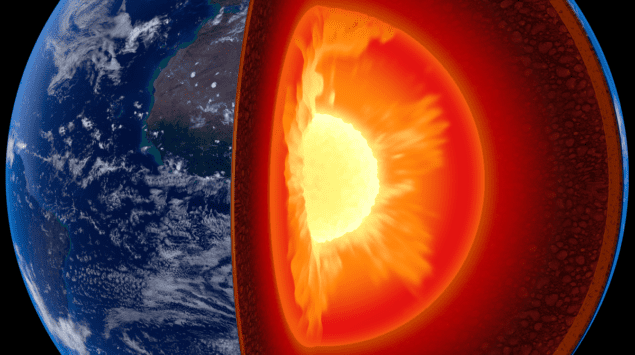
A long-standing debate about the structure of solid iron at the centre of the Earth looks set to be reignited following new laboratory tests carried out by scientists in the US. The researchers say their results imply that iron crystals in the Earth’s inner core have a body-centred cubic (BCC) arrangement – in contrast to the hexagonal-close-packed (HCP) structure pointed to by many previous results.
Scientists have good evidence that the exceptionally high pressures that exist in Earth’s inner core dictate that the iron there is solid – in contrast to the molten iron present in the outer core. That evidence comes in the form of data from earthquakes. By plotting the paths of seismic waves through the Earth, seismologists have concluded that shear waves – which cannot propagate in liquids – do not travel through the outer core but do travel through the inner one.
However, for several decades debate has raged about how the atoms in that solid iron are arranged. At room temperature and pressure, iron has a bcc lattice, which means that every atom is surrounded by eight others – four in each of the layers immediately above and below it. But at higher pressures, iron atoms form the slightly tighter hcp structure, such that each atom has 12 neighbours – six in its own layer and three in those above and below it.
Diamond anvils
The situation at very high temperatures and pressures, however, is not well understood. Several experiments using iron heated inside high-pressure diamond anvil cells have shown that here too the structure is hcp. These experiments involve squeezing tiny samples of iron between the tips of diamonds, heating them with a laser beam and at the same time illuminating them with X-rays from a synchrotron source to determine the crystal structure from the X-ray diffraction pattern.
In fact, according to Guoyin Shen of the Carnegie Institution of Washington, most Earth scientists are persuaded that the iron in the inner core does in fact have a hexagonal-shaped lattice. “Within the community,” he says, “many think that it is a done deal – that the structure of iron seems to be hcp.”
Seismic anisotropy
There is a problem, however. Seismologists have established that shock waves from earthquakes travel more quickly through the Earth when they go from pole to pole than when they go along the equator. This “anisotropy” ought to be reflected in the structure of the iron, such that it is significantly more elastic at right angles to its atomic layers than it is parallel to them. In other words, that the spacing, and hence the give, between adjacent layers should be significantly greater than that between neighbouring atoms within the same layer. But theorists have calculated that very high temperatures should flatten hcp iron – making the difference in spacing too small to account for the seismic anisotropy.
In the latest research, Shen and his Carnegie colleagues Ross Hrubiak and Yue Meng show experimentally that the inner core iron might in fact have a bcc structure. They did so after realizing that the diamond cell experiments could be giving misleading results. These “in-situ” measurements provide X-ray diffraction patterns of the iron during the brief period that it is heated by the laser. But because the X-ray beam is not much narrower than the hot spot created by the laser there is a chance, says Shen, that the high-temperature data become “contaminated” by data from regions at lower temperatures.
To try and get round this problem, Shen and co-workers have developed an alternative technique in which they compress samples of iron in a diamond anvil cell but this time take X-ray diffraction images before, during and after each heating pulse. Using the HPCAT beamline at the Argonne National Laboratory’s Advanced Photon Source, they find that at relatively low pressures and temperatures the diffraction patterns are as would be expected from hcp-iron grains.
Two distinct axes
However, once the group raised the pressure above about 106 atm and the temperature well above 4000 K it discovered that the crystal grains became oriented along two distinct axes. Because the grains’ orientation might be preserved across phase transitions, the researchers say that this “bi-axially aligned microstructure” is evidence that the iron adopts a bcc structure at high temperatures and then transforms back into hcp when it cools down.

The Earth – for physicists
Buoyed by these results, Shen and colleagues then carried out fresh measurements at inner-core like conditions. They realized that, as they and other groups had found previously, many of the spots in the diffraction pattern would be due to hcp-iron. But they predicted that they should also see one spot due to bcc-iron in a particular orientation – and say that they have observed it. “In earlier experiments we considered that diffraction spot as noise,” says Shen, “but now it becomes evidence that supports our view.”
Shen acknowledges that others in the field are likely to be sceptical, given potential doubts about his group’s interpretation of the microstructure data and the fact that it has so far only seen one family of diffraction planes that it can attribute to bcc-iron. He says that he and his colleagues are now focused on trying to improve the in-situ measurements, either by reducing the X-ray spot size or increasing the X-ray energy to boost chances of finding more diffraction information.
The research is reported on the arXiv server.