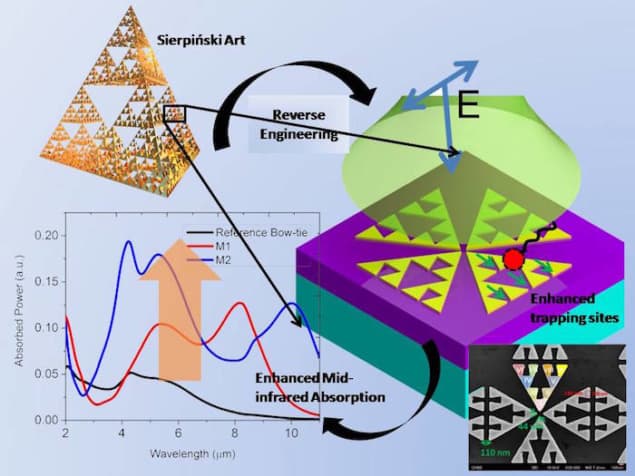
Fractals are becoming popular for when it comes to designing microwave and radio-frequency antennas thanks to their “self-similarity” that allows the antenna to better collect and focus broadband, multi-frequency light. Researchers at the National University of Singapore have now made a molecule sensor from a modified Sierpinski triangle that works in the mid-infrared range. The device could be used to profile the fingerprints of various biological elements, such as cells and protein monolayers.
Researchers have recently begun to make use of fractal patterns to manipulate surface plasmons, which are quantized collective oscillations of conduction electrons on the surface of metallic nanostructures that interact strongly with light. Such strong interactions allow the plasmons to concentrate light into subwavelength volumes, well below the diffraction limit of light. The applications are many: sub-diffraction focusing, transparent metallic electrodes, improving photovoltaic efficiency, and enhancing molecular fluorescence, to name but a few.
Modified Sierpinski fractal model
In their work, researchers Chengkuo Lee and Dihan Hasan in the Department of Electrical and Computer Engineering and the Center for Intelligent Sensors and MEMS at the NUS studied a Sierpinski fractal model made of gold and chromium.
“Our original goal was to investigate the properties of this fractal structure once we had tailored its dimensions for mid-infrared sensing,” explains Hasan. “We then came up with the idea of modifying the model slightly to drastically improve its sensing properties in this spectral range.”
The Sierpinski fractal is an equilateral-triangle-shaped fractal with intrinsic “self-similarity” – that is, its constituent triangles are repeated at smaller scales (or “orders”). Self-similarity in electromagnetic structures is important for miniaturizing the size of a device so that it can focus light at a particular frequency. In our work, we slightly modify the existing fractal model without destroying its self-similarity,” says Hasan.
Bow-tie nanostructures
The Sierpinski fractal is particularly suitable for merging with bow-tie nanostructures, he adds. These structures are among the best at manipulating localized surface plasmon resonances and enhancing light fields the most thanks to a “lightning rod” effect at their sharp tips that allow them to act as nanoantennas. These antennas possess “plasmonic modes” that can be tuned to resonate with the optical transitions in molecules nearby. It is these plasmonic modes that increase the coupling between light emitted by neighbouring molecules and the antenna, and means that they can be used as sensors.
Sierpinski fractals have already been used a key element in many high-performance plasmonics applications, such as nanolithography, ultralow power optical trapping, and plasmonic photography film for high density data storage. The problem is that it is difficult to make a pure Sierpinski fractal because of geometric differences at the junctions of the fractal components. What is more, previously-fabricated fractals did not fully exploit the lightning rod effect of nano-bow-ties.
No need for molecule functionalization
Lee and Hasan have now used advanced electron beam lithography to precisely tune the geometric structure of these fractals. By improving their architecture, the researchers have found that the structures can enhance light fields in the mid-infrared range (3000 to 8000 nm). The way the components are arranged also maximizes the lighting rod effect of the nano-bowtie/fractal patterns.
“Conventional resonant sensing focuses mostly on the visible part of the spectrum,” explains Hasan. “Here, we investigate sensing in the mid-infrared spectral range, which is the range in which many biological molecules absorb light.”
Probing these molecular absorptions does away with the need for functionalizing the biological molecules so that they can be picked up the sensor.
Towards on-chip molecule sensors
“Sensing in the infrared will also allow us to more reliably discriminate sequential events occurring in a biological system,” he adds. “And the multispectral fractal platform we describe will ultimately allow for high-throughput multiplexed detection of various molecules on a single platform. This should improve the signal-to-noise ratio of such imaging at multiple wavelengths of interest.”
The broadband and enhanced light absorption made possible by the modified fractalization is promising for sensing biological molecules at mid-infrared wavelengths, he tells Physics World. “But that is not all: the enhanced light-matter interactions that come about thanks to the increased number of light-absorbing ‘hotspots’ also makes the device more sensitive in the optical range. Here, light could be efficiently converted into heat for on-chip electronic readout, something that will help overcome one of the major limitations of mid-infrared sensors – their bulky size.”
The team, which reports its work in the IOP journal Nano Futures 2 025005, says that is now busy working on integrating the platform with 2D materials to make on-chip molecule sensors. “We are actively studying the thermoelectric properties of various 2D materials to this end,” adds Hasan.