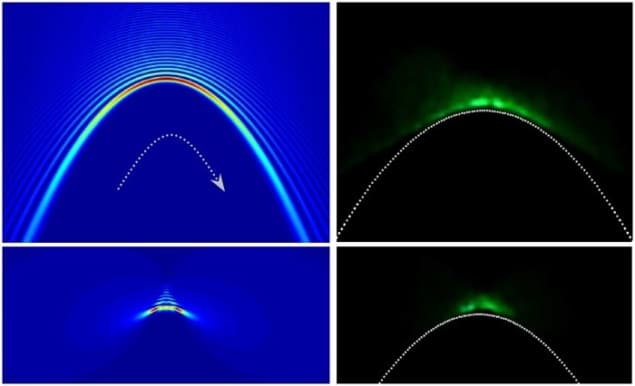
Five years ago physicists showed that certain kinds of laser beam can follow curved trajectories in free space. Such counterintuitive behaviour could have a number of applications, from manipulating nanoparticles to destroying hard-to-reach tumours. But before this bizarre effect could be put to good use, researchers were faced with the challenge of how to bend the light through large enough angles to be useful. Now, two independent teams have solved this problem – and claim that the bending of sound and other kinds of waves could be next.
The concept of self-bending light was inspired by quantum mechanics and the realization in 1979 by Michael Berry and Nandor Balazs that the Schrödinger equation could support “Airy” wavepackets of particles, which accelerate without an external force. Then in 2007, Demetrios Christodoulides and colleagues at the University of Central Florida created the optical equivalent of an Airy wavepacket. This is possible because the equation describing paraxial beams – beams in which the constituent rays all travel almost parallel to the direction of the beam’s propagation – is mathematically identical to the Schrödinger equation once several parameters are interchanged, such as mass and refractive index.
The Florida team generated a specially shaped laser beam that could self-accelerate, or bend, sideways. The researchers did not bend the laser beam as a whole but rather the high-intensity regions within it. To do this they passed a centimetre-wide ordinary laser beam through a device known as a spatial light modulator that adjusted the phase of the beam at thousands of points across its width. Rather than acting like a lens and focusing all of the beam’s constituent rays to a single point, the modulator instead changed the relative phase of the rays such that their interference produced a region of maximum intensity that curved sideways in the shape of a gentle parabola across the beam as it propagated forward, along with a number of fainter regions on one side.
Intriguing characteristics
In addition to this self-bending, the beam’s intensity pattern also has a couple of other intriguing characteristics. One is that it is non-diffracting, which means that the width of each intensity region does not appreciably increase as the beam travels forwards. This is unlike a normal beam – even a tightly collimated laser beam – which spreads as it propagates. The other unusual property is that of self-healing. This means that if part of the beam is blocked by opaque objects, then any disruptions to the beam’s intensity pattern could gradually recover as the beam travels forward.
A limitation of the Florida work, however, is that Airy beams can only be bent through relatively small angles up to about 15 degrees. This means that they cannot provide the sharp turns needed for manipulation on the micron or nanometre scale.
But then in April this year, Mordechai Segev and colleagues at the Technion-Israel Institute of Technology derived a set of general solutions to Maxwell’s equations showing that a non-diffracting non-paraxial beam should exist and that it should accelerate in a circle. A month later, two teams produced such beams in the lab – each bending light a 60-degree arc. One team was led by Xiang Zhang of the University of California, Berkeley in the US and the other by John Dudley of the University of Franche-Comte in France.
Not just circular motion
Now, two independent teams have shown, both theoretically and experimentally, that non-paraxial acceleration along trajectories other than a circle is possible. One group is led by Berkeley’s Zhang and it studied both elliptical and parabolic motions via analytical and numerical 2D scalar analysis. The other team is led by Florida’s Christodoulides and it considered elliptical motion using numerical 3D vector analysis. In the experiments, both groups used continuous-wave lasers, with a wavelength of 532 nm for Zhang’s group and 633 nm for Christodoulides’ group, shining them through spatial light modulators with phase variation calculated using special computer programs. In both cases, the groups were also able to bend the light through about 60 degrees.
According to Berkeley group member Peng Zhang, these latest studies could lead to a number of practical applications. These include particle manipulation and the burning of curved channels through air to guide plasmas for remote sensing. He also says they could be useful in medicine, allowing doctors, for example, to image or destroy a tumour behind an organ without destroying that organ. “The self-healing of the beam would be very useful,” he adds, “because it would allow you to send energy deep into tissue even with obstacles in the way.”
In addition, Xiang Zhang says that the approach can be generalized to any other kind of wave system, such as matter waves, electron waves or acoustics. In fact, he points out, his group is investigating the bending of sound waves. He believes that it should be possible to transport sound energy around corners by manipulating the phase of acoustic waves with a device equivalent to a spatial light modulator.
Ingenious, but not new?
Jérôme Kasparian of the University of Geneva in Switzerland, who was not involved in the latest work, is enthusiastic, explaining that the two groups have “elaborated a general framework to describe and therefore predict” large-angle bending of light. However, Michael Berry of Bristol University in the UK, is less so. He believes that the authors do not make it clear that in their experiments they are not bending light rays themselves but the rays’ envelopes, or “caustics”. “The technical details in these papers are ingenious and interesting to specialists, and I hope the renewed emphasis will lead to applications,” he says. “But while the papers are technically interesting, they are unsurprising because they contain no fundamental new idea.”
The research is described in two papers published in Physical Review Letters.