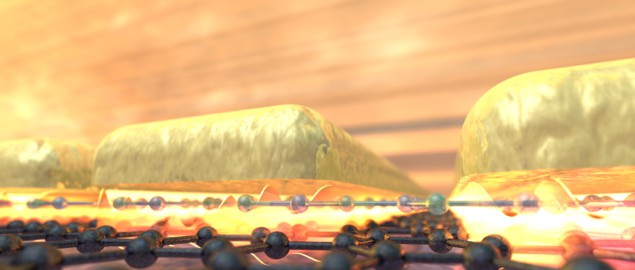
Light has been confined to a gap just one atom wide by an international team of researchers. While their work is very preliminary, it could find a range of applications including the development of tiny but powerful lasers and the extreme miniaturization of devices that use light to transmit and process information.
Photonic devices that use light pulses to transmit and process information offer important advantages over conventional electronics. Optical pulses travel faster through optical fibres and suffer much lower losses than electrical pulses sent along metal wires – and multiple signals can be sent down the same fibre without interacting with each other.
However, miniaturizing components to create nanophotonic devices remains an important challenge. Whereas electronic transistors less than 10 nm in size can be made, optical fibres are best suited for transmitting infrared light with a wavelength of about 1550 nm. Normally light cannot be confined in spaces smaller than its wavelength and so this puts a lower limit on how small photonic devices can be made.
Excited electrons
Particle-like collective excitations of electrons on the surface of a metal – called surface plasmons — could offer a way forward. Plasmons in a narrow gap between two metal surfaces can couple to a photon of light to create a surface plasmon polariton (SPP). This is a photon-like entity that can be confined in regions much smaller than the wavelength of the original photon. What is more, when the SPP reaches the end of the confining gap, the photon can be re-emitted. Another benefit of using plasmons is that they offer new ways for photons and electrons to interact, which could lead to new types of optoelectronic devices.
There are limits, however, on how narrow the gap can be. Below about 15 nm, the electric field of the confined light penetrates below the metal surface, exciting plasma oscillations that dissipate energy in a process called Landau damping. An alternative is to excite plasmons in graphene, which is only one atom thick. However, this has previously required patterning the graphene into nanoribbons: “When you pattern graphene, you have to etch it away where you don’t want it,” explains Frank Koppens of the Institute of Photonic Sciences in Barcelona. “You get rough, non-crystalline edges, and those affect the electronic and optical quality.”
Koppens and colleagues in Spain, France, Portugal and the US have now taken a different approach to using graphene. They begin with a single, crystalline sheet of graphene, that they cover with a layer of insulator. Then they add a periodic array of gold bars on top of the insulator. By shining an infrared laser on to the top of the structure, the researchers were able to excite plasmons in the graphene through the electric field in the tiny gap between the gold bars and the graphene layer. By patterning the external environment rather than the graphene, says Koppens, the researchers could neatly sidestep graphene edge effects.
Plasma frequency
The team experimented with different thicknesses of insulator and found that, down to about 3 nm thickness, the plasmons stayed well confined in the gap and did not penetrate significantly into the gold. Even when the gap was reduced to an atomic layer of boron nitride just 0.7 nm thick, the electric field leaking into the metal did not lead to Landau damping. The researchers believe this is because the resonant frequency of the graphene plasmons is much smaller than the plasma frequency in the metal, so even when the electric field spreads into the metal, it does not excite oscillations or dissipate energy.
In the near-term, says Koppens, the research could offer significant interest for enhancing nanoscale light-matter interactions, allowing much more powerful on-chip infrared emitters and possibly infrared lasers. The researchers also plan to investigate the possibility of plasmonic interconnects for electrical signals: “We have shown already that you can efficiently go from plasmon to electron, but we want to show also that you can go from electron to plasmon,” he says.
“This work is very exciting to me because, ideally, it provides a playground for how we can transmit and modulate light in an atomically thin waveguide structure,” says nanophotonics expert Nicholas Fang of Massachusetts Institute of Technology, who was not involved in the research. He cautions, however, that much work remains to be done before viable devices can be made.
“Massive paradigm shift”
Graphene expert Andrea Ferrari of the University of Cambridge agrees: “If we take this to its most extreme and futuristic implication, then we’re opening a new field where you could have light-driven optoelectronics at a scale similar to electronics: that would be a massive paradigm shift in technology,” he says. “I’m not saying that tomorrow or even in 20 years we’re going to have that, but even if it never goes to practical applications, the conceptual result is it. If you asked me or anybody else a few years ago, everybody would have said this was completely impossible.”
The research is described in Science.