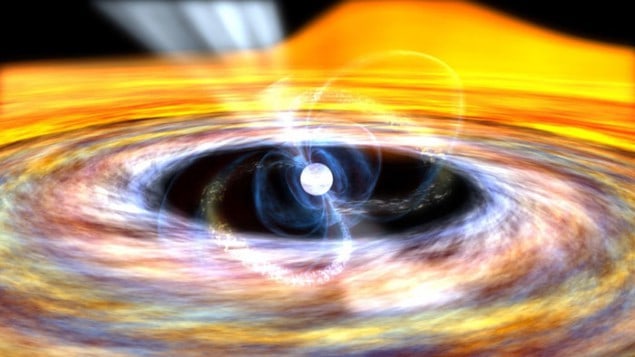
A researcher in Germany has revealed how “millisecond pulsars” – neutron stars with rotational periods ranging from 1–10 ms – slow over time. By exploring how a pulsar behaves when it stops accreting matter from a donor star, Thomas Tauris from the University of Bonn has shown quantitatively that it is the expansion of the pulsar’s magnetic field that helps to slow the star’s rotation. The finding may help astronomers to determine the age of radio millisecond pulsars, which is usually calculated based on the rate at which the pulsars’ rotation slows.
Pulsing stars
Neutron stars are highly compact remnants from exploding stars known as supernovae. As they retain most of the original star’s angular momentum but have much smaller radii, neutron stars rotate at very high speeds when they are formed. They also appear to pulse as they rotate, just like a lighthouse beam. The pulse arises from interactions between an electrical field created by the rotational energy of the neutron star and the star’s very strong magnetic field, which create an electromagnetic beam emanating from the poles of the magnetic field.
Pulsars can glow for 50 to 100 million years after the original explosion, emitting radio waves until they run out of energy and go dark. But for pulsars orbiting with companion stars, this does not spell the end. As the companion reaches the end of its hydrogen-burning phase, it begins to expand, allowing the neutron star to lift material from its surface. The filched plasma effectively breathes new life into the neutron star. The charged particles of the plasma get caught in its magnetic field and are funnelled along the magnetic field lines toward the poles. Heating through friction and impact with the pulsar’s surface causes the plasma to emit X-rays, which emanate most strongly from the magnetic poles.
Hidden transitions
As the mass moves in towards the star’s surface, the plasma also causes the star to spin faster, like a figure skater pulling in their arms. But when the companion star has given off all its envelope material, the pulsar begins to slow and emits radio waves instead of X-rays. Indeed, the 13 known accreting X-ray millisecond pulsars have an average rotation period of 3.3 ms, whereas the 200 known millisecond pulsars that emit radio waves – with spin periods of less than 20 ms – have a slower average period of 5.5 ms.
But little is known about what happens to these pulsars during this transition from emitting X-ray to emitting radio waves. Because the radio-emitting millisecond pulsars were once X-ray emitters, Alessandro Patruno of the University of Amsterdam in the Netherlands, who was not involved in the current work, says it is like accelerating a spinning top only to let it go and find it abruptly spinning more slowly. “For this to happen something must have slowed the rotation after you finished accelerating the spinning top,” he says. That braking seems to occur as the flow of matter from the donor star gradually shuts off.
Hit the breaks
What Tauris has done is to show that as the flow of plasma from the companion star tails off, the star’s magnetic field expands outwards to about 100 km, or roughly 10 times the neutron star’s radius. “The magnetosphere acts like a long lever arm, amplifying the effects of the last plasma,” explains Tauris. This means that interactions between the magnetic field and the plasma become magnified. For example, the field might blast away some of the matter away, instead of accreting it, and these interactions cut the star’s rotational energy by as much as half.
Fred Lamb, an astrophysicist at the University of Illinois, Urbana-Champagne, calls Tauris’s finding “a significant advance in our understanding of how accreting millisecond X-ray pulsars become rotation-powered millisecond radio pulsars”. He adds that while importance of this phase has been known for many years, “until this work by Tauris, no calculations modelling this phase had been performed”.
Synchronizing clocks
This slowing mechanism also sheds new light on the question of why radio millisecond pulsars seem so much older than their companions. After giving up most of its gas, the companion star can no longer burn hydrogen, but it is still hot – a white dwarf. The temperature of the white dwarf gives one measure of a binary system’s age. As a pulsar’s age is calculated based on how much its rotational period slows, this unexpected slowing leads to the age discrepancy.
In millisecond pulsars, Tauris says this method gives “ridiculous” ages such as 15 billion years – longer than the age of the universe. The dramatic deceleration as the neutron star is cut off from its donor can explain why these stars often appear much older than their companions. “There’s only one reliable clock and that’s the cooling of the white dwarf,” says Tauris.
Tauris acknowledges that the number of X-ray millisecond pulsars he could consider is relatively small as they were only discovered a decade ago. More observations of these stars, to be made with current and future X-ray satellites such as NASA’s Rossi X-ray Timing Observer, will put Tauris’s picture to the test.
The research is reported in Science.