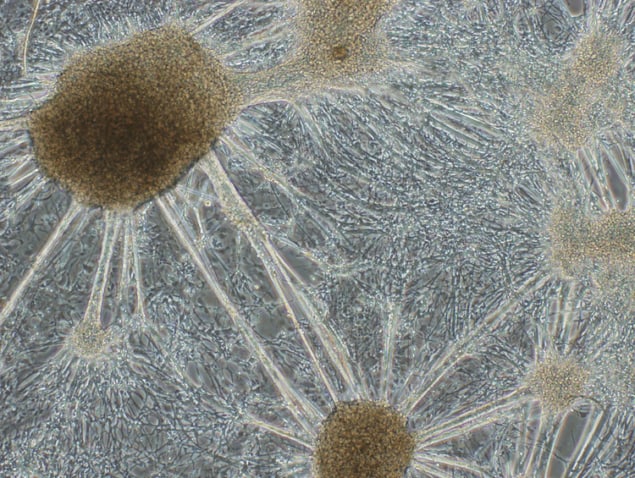
Self-organized and complex neural network activity has been observed in cerebral organoids – artificially grown 3D tissue cultures that resemble the cerebrum of the human brain. These organoids could help advance our understanding of various brain functions such as memory and intention as well as the fundamental mechanisms underlying neuropsychiatric diseases.
Recent advances in stem cell technology have allowed researchers to construct cerebral tissue from human pluripotent stem cells (hPSCs) in 3D. Cerebral organoids have the same architecture and physiology of the cerebrum, which is the largest and most complex tissue in the brain, comprising assembled activated cells organized in neural networks. The good thing about these organoids is that they mimic cerebral development and can thus be used as a substitute for the cerebrum, not only to model its development but also cerebrum-related diseases such as microcephaly, glioblastoma and Timothy syndrome, to name just three.
Researchers led by Jun Takahashi of the Center for iPS Cell Research and Application (CiRA) at Kyoto University in Japan have now imaged synchronized and unsynchronized activity in the networks and connections between the individual neurons of cerebral organoids they grew in the laboratory. Synchronized neural activity is responsible for various brain functions, including memory.
Mimicking the developmental process of the cerebrum
The researchers grew active cerebral organoids from a ball of hPSCs by placing the cells in a culture medium that mimicked the environment necessary for cerebral development. They generated the organoids via the SFEBq (serum-free floating culture of embryoid body-like aggregates with quick reaggregation). This method was pioneered by the stem cell pioneer Yoshiki Sasai and is now one of the most common procedures for producing this tissue.
“One of the most interesting properties of these organoids is that they actually recapitulate the developmental process of the cerebrum in their shape,” explains team member and lead author of this new study, Hideya Sakaguchi. “Their layered structure is very beautiful and looks just like actual brain tissue.”
The team cultured the organoids for 70-100 days, dissociated them into single cells and then disseminated them into another culture dish. The disseminated cells created neuronal networks in a self-organized manner, says Sakaguchi.
Synchronized bursts
The researchers then measured the activity of calcium ions (Ca2+) of all of the neuronal cells in a field and built maps of patterns reflecting the activity of over 1000 cells. The concentrations of Ca2+ fluctuate during neuronal activity and abnormal Ca2+ signals are thought to be implicated in brain disorders, such as epilepsy and Alzheimer’s disease. By imaging the intracellular calcium dynamics of the network in this way, Sakaguchi and colleagues showed that the cells in the networks show synchronized bursts of activity with some spontaneous individual activity.
The researchers used a popular technique to measure neuronal activity in their organoids that relies on using confocal or multi-photon microscopy to detect the fluorescence produced when Ca2+ ions bind with calcium indicators in neurons.
“We chose this method because it can be used to detect the shape of a neuronal network and relate it to its function,” explains Sakaguchi. “Combining this technique with our high-content analytical approach allows us to visualize synchronized and non-synchronized network clusters and divide the synchronized ones into several smaller clusters, to provide us with information on cell distribution.”
Ethical concerns
Growing artificial brains in the lab is a sensitive area of research to say the least and many have raised concerns about the ethical issues – for instance, regarding the neural function of these cerebral organoids. Since these structures mimic the developmental process of the brain, could they also acquire mental activities such as consciousness in the future? Takahashi and Sakaguchi think not – simply because the organoids lack sensory input from their surrounding environment. The famous “brain in a vat” thought experiment, proposed by the philosopher Hilary Putnam, is thus still safely in the realms of science fiction.
“Consciousness requires subjective experience, and cerebral organoids without sensory tissues will not have sensory input and motor input,” says Sakaguchi in a press release issued by CiRA. “However, if cerebral organoids with an input and output system develop consciousness requiring moral consideration, the basic and applied research of these cerebral organoids will become a tremendous ethical challenge.”

Laser bioprints stem cells
Three main application areas
Organoid research such as ours will have three main application areas in the future, he tells Physics World. The first is in drug discovery. “Our analysis technique could allow us, for instance, to detect the minimum dose of a drug that causes abnormal neural network activity – something that could come in useful for pharmaceutical companies wishing to replace conventional drug evaluation methods that rely on animal models.”
The second area of research is in the modelling of neuropsychiatric disorders (especially complex, currently untreatable ones) and better understand those that degrade neuronal activity by analysing cell activity patterns, he says. Ultimately, this could lead to reliable psychiatric disease models using patient-derived induced pluripotent stem cells (iPSCs).
The third area involves regenerative medicine – for example, attempting to improve loss of brain function caused by specific diseases such as cerebral haemorrhage, cerebral infarction or physical trauma.
Full details of the research are published in Stem Cell Reports.