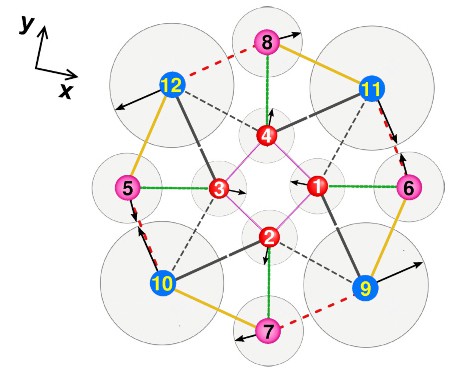
The complete magnetic properties of the prototype molecular magnet Mn12 have been modelled, for the first time, by an international team of researchers. The calculations will be crucial for developing real-world devices from the material, as well as to study fundamental nanoscale quantum-phenomena such as magnetic tunnelling.
Single-molecule magnets, such as Mn12, Fe8, Mn4 and V15, are natural ensembles of identical, weakly interacting magnetic nanoparticles that can switch their magnetization between two states, from “spin up” to “spin down” for example. At low temperatures, the magnetic state of the molecule persists even in the absence of a magnetic field. Such a “memory effect” could be exploited to make high-density information storage devices for computing applications and in molecular electronics in general.
Mn12 is a prototype molecular magnet and as such, is also an ideal model object in which to study physical phenomena such as spin dynamics and quantum decoherence in nanoscale quantum systems. The molecule contains 12 magnetic ions with high spins, so the space taken up by these quantum states (known as the Hilbert space) is very large.
No ‘adjustable parameters’
“Our calculations prove that modern quantum-physics models can be used to study magnetic interactions in such a complicated system, from first principles,” explains team leader Mikhail Katsnelson of Radboud University of Nijmegen in the Netherlands. “Thanks to methods that we developed previously, we have now managed to analyse all the magnetic interactions within this molecule – and without any ‘adjustable parameters’.” The calculations seem to back up results from inelastic neutron scattering experiments on Mn12, he further explains.
Until now, most theoretical work on molecular magnets mainly relied on the so-called rigid-spin model, in which the whole system of interacting spins is replaced by just one big spin, with some magnetic anisotropy being introduced “manually”. However, such a description is rather simplistic and largely ignores intermolecular interactions.
Interactions are important
From their earlier work, the researchers had suspected that special kinds of magnetic interactions, known as antisymmetric exchange or Dzyaloshinskii–Moriya (D–M) interactions, could, on the contrary, play a crucial role in the physics of molecular magnets. “We have now confirmed this assumption quantitatively,” says Katsnelson.
The D–M interaction is a consequence of the spin-orbital interaction, which couples electronic orbital and intrinsic spin magnetism. It is the part of this interaction that favours perpendicular coupling of magnetic moments, rather than the normal parallel/antiparallel coupling. The interaction causes a periodic (left, right, left, right) twisting (or “canting”) in weak ferromagnets. It also appears to be responsible for the magnetoelectric effect in multiferroics – materials with both magnetic and electric ordering. The latest calculations could perhaps now inspire new experiments for detecting a weak in-plane antiferromagnetic ordering originating from the D–M interactions.
Spurred on by their new results, Katsnelson says that he and his co-workers will now be applying their model – which also takes into account Heisenberg exchange interactions and magnetic anisotropy – to other molecular magnets and nanoscale magnetic clusters.
The research is published in Physical Review B.
- This article first appeared on nanotechweb.org.