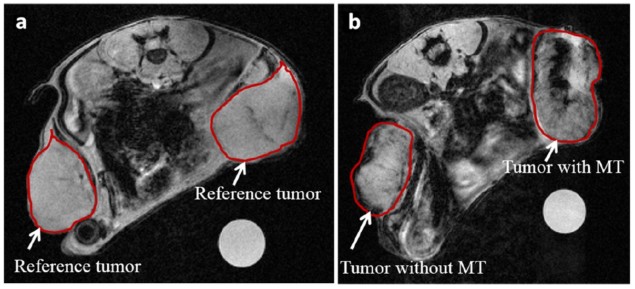
Cancer chemotherapy is well known to cause toxic side effects and so, unsurprisingly, scientists are keen to develop a targeted delivery system that avoids adverse impact on healthy tissue. One attractive targeting mechanism is to magnetically guide nanocarriers of toxic drugs to tumours using an externally applied magnetic field. Magnetic targeting is cheap, easy to handle and versatile; however, it currently lacks a quantifiable method for evaluating targeting efficiency within patients.
MRI is a non-invasive method for in vivo detection, producing 3D images proven to enable quantification of contrast agents such as gadolinium, for example. Now a French research collaboration has proposed a new method to process MR images for iron oxide quantification.
The researchers created ultra-magnetic nanocarriers and applied magnets to target the nanocarriers to colon tumours in mice. They then developed a novel quantification method based on the modification of intensity distributions found in MRI (Mol. Imaging Biol. 10 1007).
A magnetic target
Liposomes are popular nanocarriers because of their biocompatibility and versatility, but for efficient magnetic targeting, liposomes have to contain high levels of iron oxide. Researchers in the group of Christine Ménager had previously achieved high loading of magnetic nanoparticles of maghemite (γ-Fe2O3) into liposomes using the reverse phase evaporation process. Now members of Ménager and Bich-Thuy Doan’s teams manufactured these ultra-magnetic liposomes (UML) for magnetic targeting.
Iron oxide nanoparticles are good T2-weighted MRI contrast agents. Thus the researchers used dynamic susceptibility contrast MRI with a T2 timed schedule of radiofrequency pulse sequences to monitor UML distribution in mice. Initial injections of UML were used to assess the nanocarrier stability and survival time in circulation. Hepatic monitoring showed that total uptake of UML (and therefore its exit from circulation) occurred 1 hour post-injection, and from this, the UML time in circulation for efficient magnetic targeting was estimated at 30 minutes.
With experimental parameters now established, the scientists implanted CT26 murine colon carcinoma cells into the posterior flanks of Balb/C female mice. After two weeks of tumour growth, mice were put under anaesthetic and pre-injection imaging acquired.
The researchers then placed magnets over the skin of one tumour, leaving the tumour on the opposite flank of each mouse as a control. They intravenously injected UML, then after 30 minutes the magnets were removed, post-injection MR images taken and the animals sacrificed for ex vivo processing.
UML signal was observed in non-magnetic targeted tumours due to passive accumulation. To compare the two types of accumulation, the scientists calculated the pixel intensity in each tumour’s MRI slices. Intensities were compiled to give a single pixel intensity distribution per tumour, but comparing the mean signals between tumours didn’t show any differences.
Adjusting for intensity
The researchers noticed that UML presence in the post-injection images caused a shift in the intensity distribution towards the lower end, and realised that this was affecting their results. To adjust for this low-intensity shift and enable standardized comparison between time points and tumours, they calculated the percentage of pixels under the I0.25 value (0.25*(maximum intensity – minimum intensity) for each tumour in an animal.
This new semi-quantitative method was able to distinguish differences between differently treated mice and their tumours. The average I0.25 for tumours that only passively accumulated UML increased from 2.9% (in a reference tumour) to 15.3% in injected mice. Magnetic targeting, however, accumulated significantly more UML in tumours, with 28.6% under the I0.25.
Ex vivo processing of murine tumours and organs validated the in vivo MRI quantification technique. Confocal imaging of a fluorescent tag added to UML lipids confirmed that magnetically targeted tumours had higher UML presence than those without. Iron titration experiments confirmed the same result, showing a three-fold accumulation of iron in tumours with magnetic targeting.
This new method enables swift and robust analysis of non-homogenous MRI signal on any MRI system. And now they have a tool to quantify targeting efficiency in vivo, the researchers seem keen to trial drug encapsulation within the UML.
The researchers also want to evaluate the accumulation efficiency in different tumour types and suggest that their method could be applied to compare other targeting methods that utilize T2 contrast nanocarriers.