A new wave of “bionanosensors” may make it quicker, cheaper and easier to detect harmful micro-organisms, but hurdles remain before they can enter the clinical realm, as Joe McEntee reports
The microscopic world is a messy and sometimes dangerous place. Bacteria, viruses and other organisms lurk on every surface, and while most of them are harmless – even beneficial – to humans, the task of detecting and classifying the ones that aren’t has long been a cornerstone of the public health system. Whether it’s a doctor seeking a rapid, definitive diagnosis of an infectious disease, a manufacturing manager monitoring for bacterial hazards in a food-processing plant, or a government agency formulating a response strategy for bioterrorism – all are able to call on an array of tried, tested and sensitive techniques for isolating and identifying harmful micro-organisms.
This big picture might seem broadly reassuring, but a closer look reveals that these existing methods for pathogen detection suffer from significant drawbacks. Some of the more sophisticated approaches – for example, immunology-based techniques or tests to identify a micro-organism’s genetic material – require costly equipment and specialist operating staff. Others, such as the ubiquitous microbial culture, have response times of days, rather than the hours or minutes that might save lives in a crisis.
In short, there’s plenty of room for improvement, and anyone – be it an academic research team, a start-up company or an established manufacturer – who can push the technology forward stands to make significant commercial as well as clinical gains. One promising strategy for doing this revolves around a class of nanoparticles that react in detectable ways when exposed to hazardous pathogens. Although still in the early stages of development, these “bionanosensor” technologies combine rapid response times (making it possible to identify pathogens in minutes rather than hours or days) with the potential for cheaper diagnostics at the patient’s bedside, while also matching the sensitivity and reliability of conventional methods.
Proof of principle
Karen Faulds, a chemist at the University of Strathclyde, UK, is at the forefront of the burgeoning research effort on nanoparticle diagnostics. She and her team at Strathclyde’s Centre for Molecular Nanometrology are developing a bionanosensor for pathogen detection that combines silver nanoparticles with a laser-based sensing scheme called surface-enhanced Raman scattering (SERS).
The Strathclyde team’s assay uses magnetic nanoparticles to isolate bacterial pathogens en masse from the sample matrix. After adding silver nanoparticles that have been functionalized with strain-specific antibodies, the researchers then use SERS to detect in real time which classes of bacteria are present. Like conventional Raman scattering, SERS exploits the inelastic scattering of photons incident on a material’s surface. However, for target molecules attached to or near the surface of the noble-metal nanoparticles, SERS offers orders-of-magnitude increases in detection sensitivity. It also produces sharp, molecularly-specific spectra that enable researchers to discriminate between several species of pathogen in a single sample – a capability known as multiplexing.
In a series of proof-of-concept experiments, carried out in collaboration with Roy Goodacre (then at the University of Manchester, now the University of Liverpool), Faulds and her colleagues Duncan Graham and Hayleigh Kearns demonstrated the potential of the SERS/nanoparticle scheme for isolating and detecting three common bacterial pathogens (E. coli, Salmonella and methicillin-resistant Staphylococcus aureus, or MRSA) from the same sample matrix, at concentrations as low as one colony-forming unit per millilitre sample. Although this is still foundational research, the long-term clinical opportunity is clear. “The sensitivity will allow rapid detection without having to carry out lengthy culture steps,” explains Faulds. In her view, the main challenges lie in scaling up nanoparticle synthesis and functionalization, as well as engineering a single instrument to separate and detect bacteria.

Faulds and her colleagues already have other pathogen targets in their sights. In particular, they are exploring a similar approach for detecting biomarkers related to sepsis. This life-threatening condition arises when the body’s immune response to infection turns on its own tissues and organs. Because the early symptoms of sepsis can mimic other illnesses, diagnosis is often delayed until the patient is seriously ill. In these cases, it is crucial for doctors to identify the responsible pathogen quickly, so that appropriate, life-saving treatment can begin.
The group’s research programme has diversified in other respects as well. Last year, Faulds and her colleagues received a grant from the UK Biotechnology and Biological Sciences Research Council (BBSRC) to evaluate their SERS/nanoparticle sensing scheme for detecting bacteria in the genus Listeria. Listeriosis – an infection caused by ingesting Listeria in contaminated food – is one of the most severe forms of foodborne infection, with an overall mortality rate of 30%. This rises to 40% in vulnerable individuals such as patients with weakened immune systems, the elderly, pregnant women and fetuses. While outbreaks are very rare, owing to strict hygiene standards in food-processing plants, current methods for detecting Listeria are far from optimum, with the growth of a bacterial culture in an offsite lab taking up to seven days.
The SERS/nanoparticle scheme could, in principle, reduce that detection time to a few minutes. By the end of the three-year project (mid-2021), Faulds and her team aim to be able to test swabs that have been taken from food-processing areas in a factory setting. However, not everything about such tests will be entirely realistic – and that, Faulds explains, is exactly as it should be. “Fortunately for us consumers, the rigorous cleaning protocols are so good, and these areas so clean, that it is likely we will need to spike the samples to get a positive result,” she says.
As for commercial opportunities, Faulds believes they are still some way off. In addition to improvements related to the nanoparticles themselves, firms would need to develop a user-friendly device in which the detection assay and a portable SERS instrument work together in a seamless way. Even so, the Strathclyde project has already attracted support from two industry partners. Wasatch Photonics, a US optics manufacturer, is providing SERS instrumentation and know-how on the optical sensing configuration. Meanwhile, Bradgate Bakery, one of the UK’s largest sandwich manufacturers, is supplying both financial support and guidance on the testing requirements for a chilled-food production environment.
Manufacturability, scalability, stability
While academic scientists like Faulds are, for now, focused on proof-of-concept research, early-stage start-ups are refining their technologies and processes with manufacturability and scalability in mind. Among that cohort is Stream Bio, a UK venture that is eyeing up a range of bioanalytical applications for its portfolio of highly fluorescent molecular imaging probes.
The probes – which are licensed by Stream Bio, though originally developed at King’s College London – comprise a core of semiconductor light-emitting polymers and iron-oxide nanoparticles encapsulated within a water-friendly capping agent. The wavelength of light emitted by these conjugated-polymer nanoparticles (CPNs) depends on the make-up of the polymer core, with four discrete polymer building blocks providing the basis for a family of fluorescent imaging probes across the visible spectrum (475–680 nm).
Stream Bio claims it will ultimately be possible to use its CPNs to detect and quantify a range of molecular targets – pathogens, biomarkers or specific DNA sequences – by functionalizing the nanoparticles’ surfaces with different active biomolecules that attach themselves to such targets. In addition, the presence of the iron-oxide nanoparticles in the CPNs confers magnetic properties that are potentially useful in any purification and enrichment step before or during analysis.
Right now, the main applications for CPNs lie in analytical tools such as fluorescence imaging microscopy, flow cytometry and plate-based assays that are widely deployed in biomedical research and clinical applications. “The intensity of the CPN fluorescence allows for a dramatic improvement in the detection capabilities of these techniques,” explains Dermott O’Callaghan, Stream Bio’s head of product development. “That enhanced capability will also improve the performance of diagnostic tests, meaning that infections can be detected sooner – of particular importance for time-critical diseases such as sepsis.”
Mark Green, Stream Bio’s director of research, acknowledges that getting the CPNs into the clinic will be “a long road”. He and his colleagues have already taken several important steps in that direction – notably by developing a commercial manufacturing process in conjunction with the Centre for Process Innovation in Sedgefield, UK. Scaling up production was “a big challenge”, says Green, but Stream Bio is now able to make CPNs to order, while maintaining the materials’ strong optical properties, stability and relatively small particle size (70–80 nm). Additionally, using funding from a UK government body, Innovate UK, Stream Bio has started developing smaller particles and more wavelengths.
Stream Bio’s pivot to a more commercial footing is also evident in its emphasis on long-term product stability and brightness. “Since the basic materials we use – conjugated polymers – were originally designed for use in the display industry, we have a robust material platform that can withstand harsh conditions and processing,” says Green, who is also a professor of nanoimaging at King’s. “We have samples prepared well over a year ago in our scale-up process that still appear as bright as they ever were after being stored in ambient conditions.”
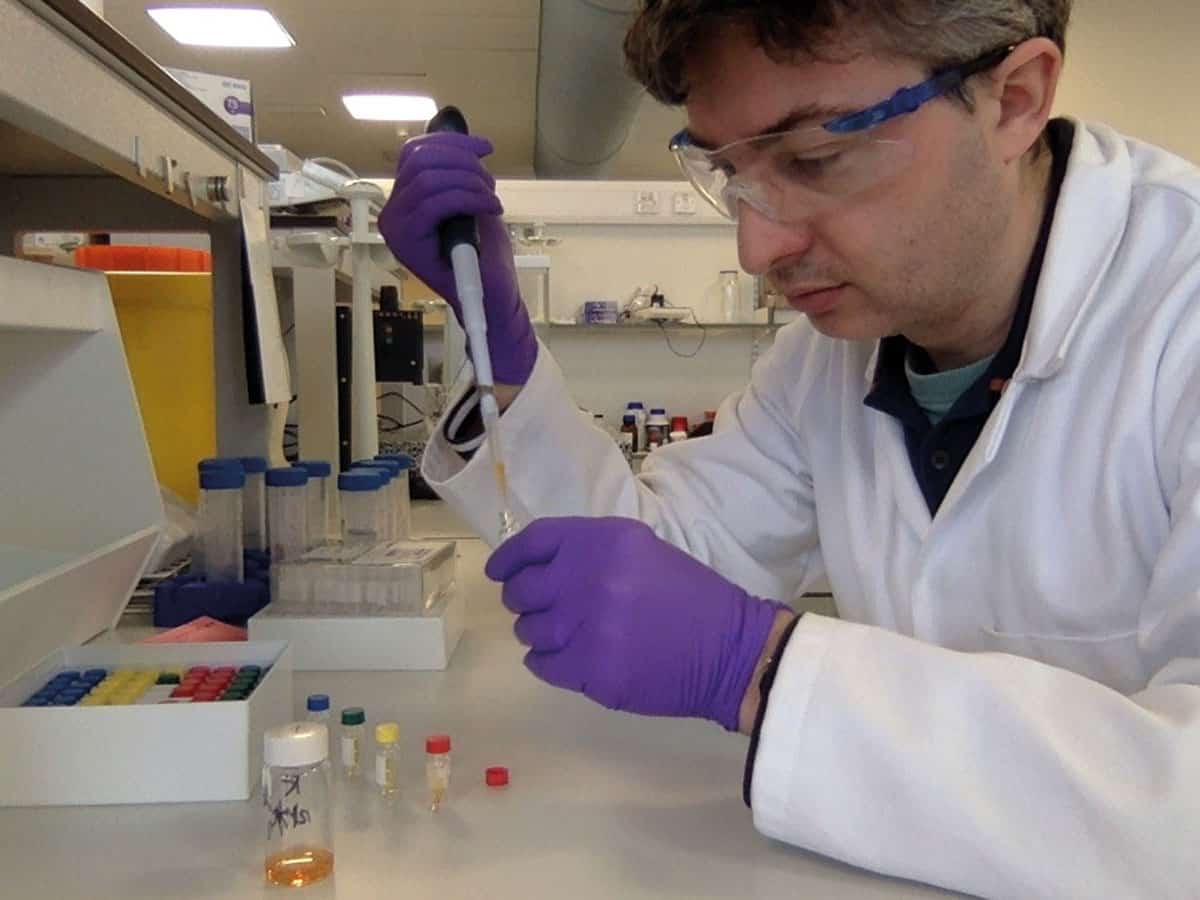
What you know and who you know
Another start-up targeting the nanoparticle diagnostics market is Bristol-based FluoretiQ. The company is currently refining its nanoparticle-based detection scheme to work on a single species of bacteria, E. coli, which accounts for around 80% of urinary tract infections. However, in the longer term, chief executive and co-founder Neciah Dorh hopes to streamline the diagnosis of all manner of bacterial infections, yielding results in minutes rather than days.
FluoretiQ’s detection scheme exploits two areas of research pioneered at the University of Bristol. First, there’s the role that carbohydrate chemistry plays in cell recognition during a bacterial infection: it’s the targeted interactions with these complex biomolecules that enable E. coli bacteria to attach themselves to the surface of cells in a human host. In the same way, when FluoretiQ’s nanoparticle probes are injected into an infected urine sample, they selectively attach to individual bacteria, making them detectable by optical methods.
“Think of our probes as a fluorescent ID badge for cell recognition,” explains Dorh, who completed his PhD in nanophotonics at Bristol’s Centre for Quantum Photonics in 2016. By measuring the fluorescence, he and his colleagues can determine what strains of bacteria are present and in what quantities. Such precise measurements are possible thanks to the other area of research FluoretiQ is seeking to exploit: enhanced quantum measurement techniques that can resolve fluorescence emissions at the single-photon level.
The team has developed both a low-cost fluorometer and proprietary data-processing techniques that can measure the photon-arrival statistics at the detector. That information is then deployed to assess whether the target fluorophore is present and whether it’s on its own or experiencing interference from other fluorescent material, such as normal metabolites.
Selectivity aside, Dorh believes that the biggest advantage of FluoretiQ’s approach is speed. The most common test for E. coli is still the microbial culture, a time-consuming and labour-intensive process. It takes two to three days for a pathology lab to grow a microbial culture from a sample of urine, after which a further series of biochemical tests is needed to home in on a definitive identification. “We’re turning all that on its head,” says Dorh. By skipping the culture-growth phase and targeting the bacteria directly, rather than via a series of steps that lead to an inferred identification, the FluoretiQ team eventually hopes to compress the time for diagnosis down to 15 minutes.
Earlier this year, FluoretiQ’s scientists got their first chance to evaluate the company’s prototype fluorometer in a real-world setting, carrying out more than 300 measurements in a National Health Service laboratory. These measurements will help inform their next stage of product development. And, like Stream Bio did, they are also working to scale up production. In December 2018 the founders brought in a synthetic chemist from the University of Bristol to help them scale up nanoprobe production (from the milligram scale up to hundreds of grams), while also focusing on yield, cost of production and reproducibility.
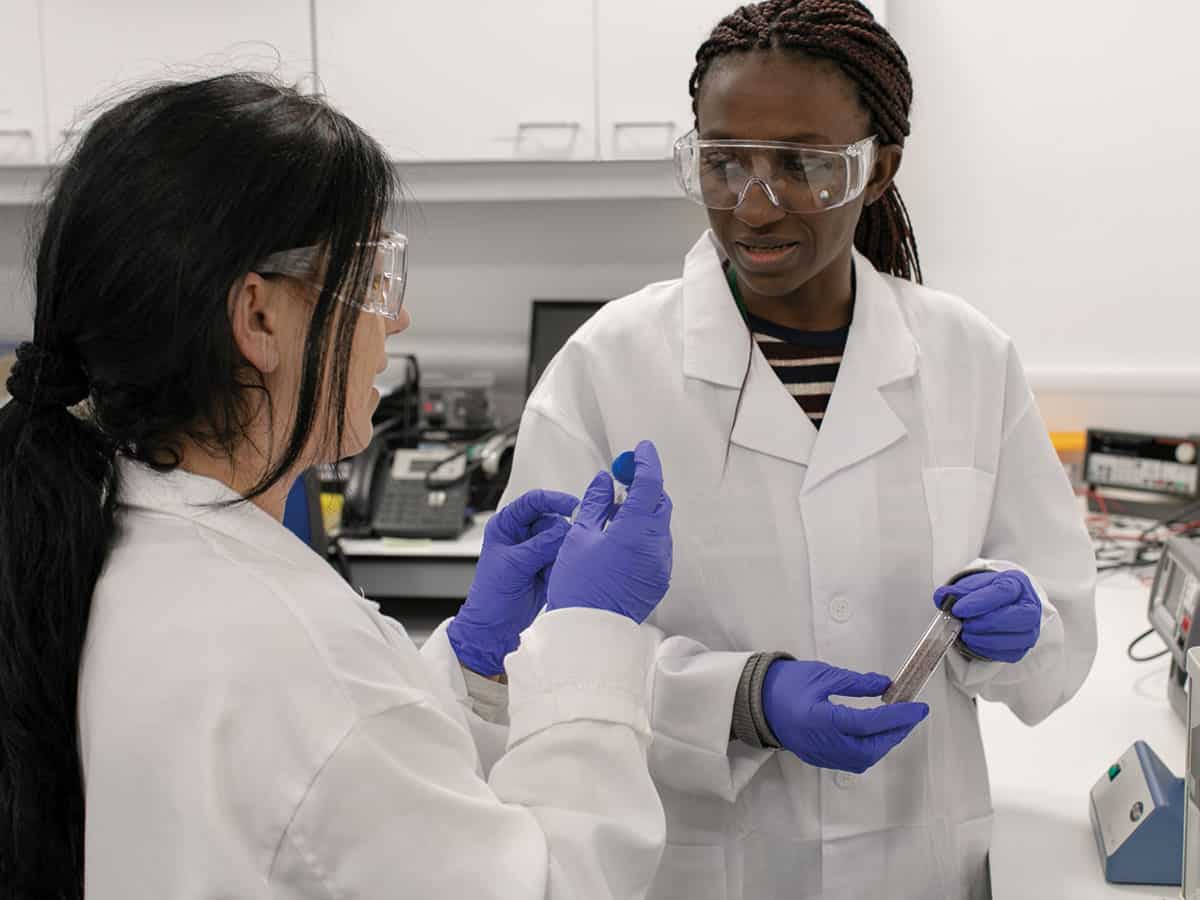
Even in these early stages, though, Dorh is already casting the net wider in order to, as he puts it, “de-risk” the next stage of commercialization. “The healthcare space is dominated by some very big companies and we’re actively seeking strategic partnerships,” he explains. “They’ll be a good fit with much of the advanced R&D we’ll have to do in the medium term – for example, around sample handling and preparation – and we’re very much open to that model of working. The next 12 months will be about finding the right partner to make sure we deliver true impact, not just in the UK but globally as well.”
Ultimately, it’s this sort of open-innovation mindset – characterized by multidisciplinary collaboration and proactive engagement with industrial and clinical partners – that starts-ups such as FluoretiQ and Stream Bio, as well as their counterparts in academia, hope to use to accelerate their R&D programmes and unlock the promise of nanoparticle diagnostics. If they succeed, a new era of cheap and easy-to-use bionanosensors beckons – saving time, saving money and saving lives.