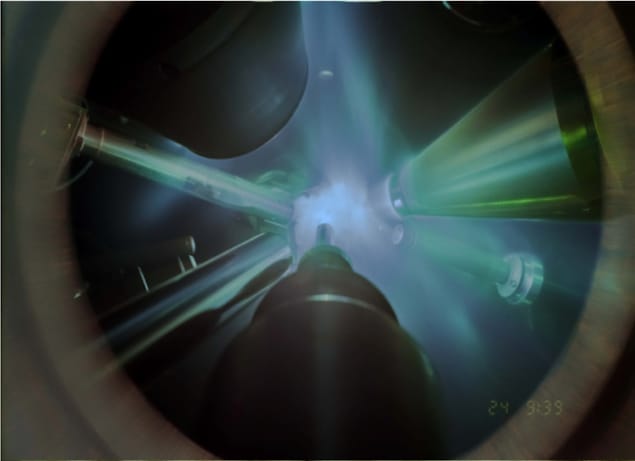
By using shock waves to simultaneously compress and heat water to pressures of up to 400 gigapascals and temperatures of 3000 kelvin, researchers at the Lawrence Livermore National Laboratory in California and the University of Rochester in New York say they have produced a new phase of solid superionic ice. The ice XVIII, as it has been named, is made up of liquid-like hydrogen ions (protons) diffusing through a solid lattice of oxygen atoms. Such superionic ice is thought to form a large fraction of the interiors of the planets Uranus and Neptune and the new work could help us better understand the structure of these icy giants and perhaps even shed more light on their complex magnetic fields.
American physicist Percy Bridgman was the first to discover five solid water phases in 1912 and we now know of more than 17 crystalline and several amorphous ice structures. This unique behaviour of water partly comes from its weak intermolecular hydrogen bonds.
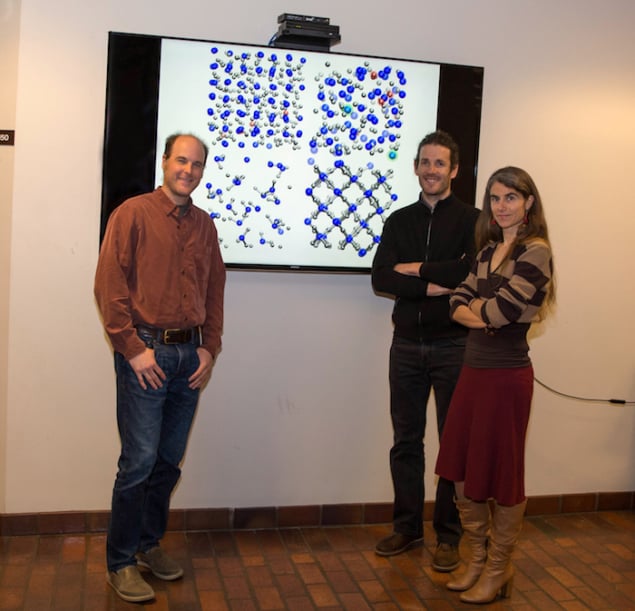
“Our research aims to explore the unusual properties of water under extreme pressures and temperatures (like those that exist deep inside planets),” explains Marius Millot, who is the co-lead author of this new study. “Unlike the various phases of water we are familiar with (liquid, vapour and ice), we were able to create and study a new exotic ice phase called superionic ice.”
Superionic ice is predicted to exist when water is subjected to more than 100 gigapascal pressures and temperatures of above 2000 K. Here, the protons diffusing through the empty sites of the oxygen solid lattice allow the ionic conductivity of water to exceed 100 Siemens per centimetre, which is almost as high as the conductivity of metals. When ice is superionic, its melting temperature increases to several thousand kelvin and new ice structures with a close-packed oxygen lattice form.
Laser-driven shock waves
Creating such high pressures and temperatures in the lab is obviously very difficult, but Millot and colleagues have now used a series of laser-driven shock waves to do this. The researchers begin by first filling small cavities created between two thin diamond disks with a tiny droplet of water (30 μm thick and 1.5 mm across). They then place this droplet in vacuum at the centre of the Omega Laser target chamber at University of Rochester’s Laboratory for Laser Energetics and use six high-power lasers to generate a series of shock waves that squeeze and heat up the droplet.
“We very carefully designed this series of shock waves so that we could access pressures of 100 to 400 GPa (1 to 4 Mbar) at temperatures of 2000-3000 K,” says Millot. “Under these conditions, water is expected to become solid and crystallize into a dense ice.”
To test this hypothesis, the researchers performed X-ray diffraction measurements on the sample just a few billionths of a second after they had the launched the shock waves. They made these measurements using a group of 16 additional high-power laser beams to deliver 8 kilojoules of light in a one-nanosecond burst onto a 250-μm spot on a 2-mm2 piece of a thin foil of iron that they placed 2 cm away from the water droplet.
Backing up previous research
“Under such intense radiation, most of the thin foil vaporizes and ionizes into a hot plasma that starts to emit X-ray photons at a very specific energy (its line emission),” explains Millot. “This flash of X-rays floods the water sample and since it has just crystallized into tiny nanometre-sized ice cubes, some of these X-rays are diffracted (deflected in particular directions, characteristic of the crystal structure adopted by the ice) and hit our image plate detectors.”
The technique allowed the researchers to confirm that the atoms are arranged in a regular lattice and that they had indeed solidified liquid water into a crystalline oxygen lattice of superionic water ice – on the timescale of just 3-5 nanoseconds.
The new result backs up previous research the researchers reported last year in Nature Physics, in which they obtained the first experimental evidence for superionic water ice at pressure-temperature conditions similar to those in the present experiments.
Slow convection
“As mentioned, these conditions are the same as those deep in the interior of Uranus and Neptune,” explains Millot. “Now that we have a clear and direct signature for a crystalline lattice in superionic water ice, we argue that this ice should not flow like a fast swirling liquid, such as the fluid iron outer core of the Earth, but that it should flow instead like the Earth’s mantle, which is made up of solid rocks yet convects on geological timescales.”
Our observation could help us better understand the internal structure of the icy giants, and indeed their water-rich exoplanet cousins, he tells Physics World. It could even shed more light on the complex magnetic fields on these planets. According to measurements made by NASA’s Voyager 2, these are thought to be quite different from the simple dipolar fields on Earth and other planets.
BCC to FCC
The X-ray diffraction data also allows the team to measure how the water ice compresses under these extreme conditions.
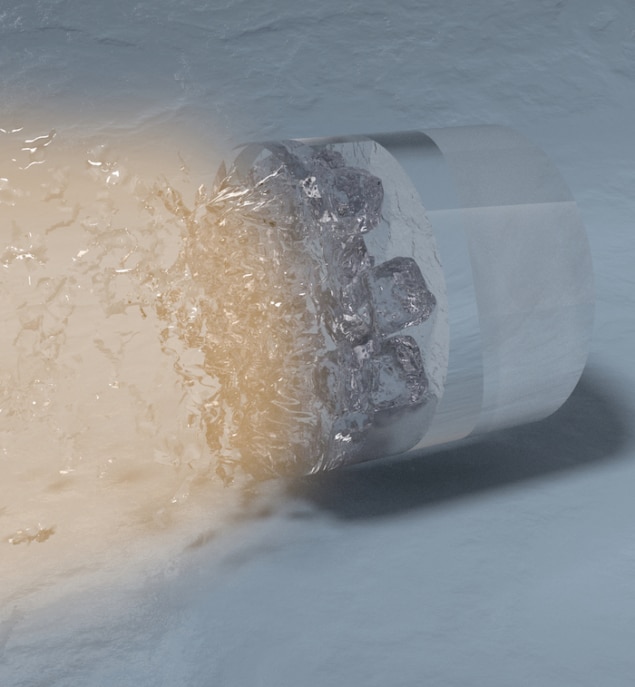
“We did our experiments over a broad pressure range of between 1 and 4 Mbar and measured how compressible water is at these pressures,” says Millot. “It turns out that the observed behaviour agrees with theoretical predictions, but when we increase the pressure above 250 GPa near 2000 K, we find that ice undergoes a change in its atomic structure to adopt a more compact arrangement of the oxygen atoms.
“In fact, it goes from being a body-centred-cubic ice phase (probably ice X) to a novel face-centred-cubic superionic ice phase. Put simply, it goes from being a Rubik-cube-like lattice with oxygen ions at each corner of the cubes and one atom at the centre of each cube to one in which the oxygens occupy all the corners and the centre of each face of the cubes.”
Towards ice XIX
This is the first time that such a crystal structure has been observed for ice, so the researchers have dubbed it ice XVIII. Since this new phase is predicted to exist only at the high temperatures at which ice should become superionic, the result is further proof that water ice indeed becomes superionic under these conditions, says Millot.

Formation of other-worldly ‘hot ice’ observed for first time
Water isn’t the only molecule that could be studied using the technique described in this work, he adds. “We will now be able to study the thermodynamics and kinetics of crystallisation into new dense ice phases in similar molecular fluids. This is exciting because many materials have been predicted to have exotic new bonding states at extreme pressures and temperatures and we could now investigate these states.”
The team, reporting its results in Nature 10.1038/s41586-019-1114-6, says that it is now searching for ice XIX.