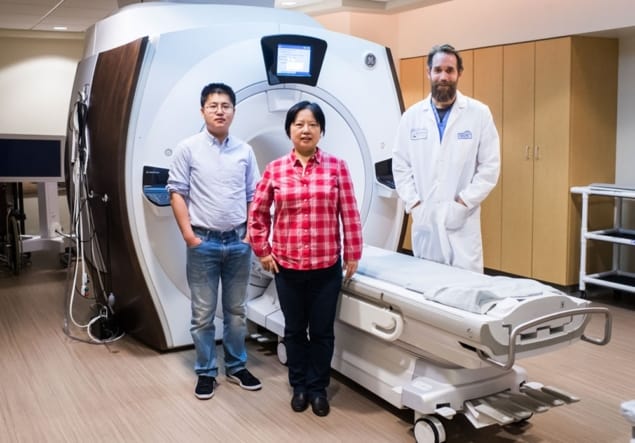
MRI is a mainstay of clinics around the world. It is an imaging technique that provides unparalleled anatomical and pathological information while also avoiding exposure to ionizing radiation.
MR does have some limitations, however. MR signal arises from a small fraction of protons within the anatomy being imaged. It is therefore difficult and expensive to separate MR signal from noise. The protons are also excited by radiofrequency (RF) radiation, which, while non-ionizing, dissipates energy as heat within tissues. As a consequence, for patient safety reasons, there are regulatory limits on the maximum RF power at which clinical MR scanners can operate.
A research team led by Xin Zhang of Boston University has developed a new type of MR signal receiver coil that amplifies the MR signal acquired during image acquisition without increasing the RF power transmitted during scanning. The approach could open the door to quicker, higher quality MR imaging, while maintaining the high levels of patient safety associated with MR scans (Adv. Mater. 10.1002/adma.201905461).
Going nonlinear
An MR scan can be thought of as an orchestra of RF pulses interspersed with the expertly timed application of magnetic field gradients. It is the rapid switching of gradient directions that creates an obnoxious racket during an MR examination. The RF pulse is used to excite the patient’s protons into coherent motion. Once the protons are in motion, the externally applied RF can be switched off, and the protons produce RF radiation of their own accord. It is this that is recorded as the MR signal.
Metamaterials, composites with properties not readily reproduced in nature due to their structure, are not new. Linear metamaterials have been used to improve the signal-to-noise ratio (SNR) in MR images by amplifying the RF pulses. The problem with linear metamaterials is that RF amplification occurs during both excitation and signal acquisition, meaning that more of the energy from the RF field is absorbed. Over long periods of time, this RF absorption can pose a safety risk, thus limiting the utility of linear metamaterials.
To address this, Zhang and colleagues produced a nonlinear metamaterial by coupling a coil of wire with a small break in the circuit, known as a split ring resonator, to a linear metamaterial. They used a 3D-printed scaffold to structure the new composite material. Using a split ring resonator allowed the researchers to control the response of their nonlinear metamaterial to applied electromagnetic fields.
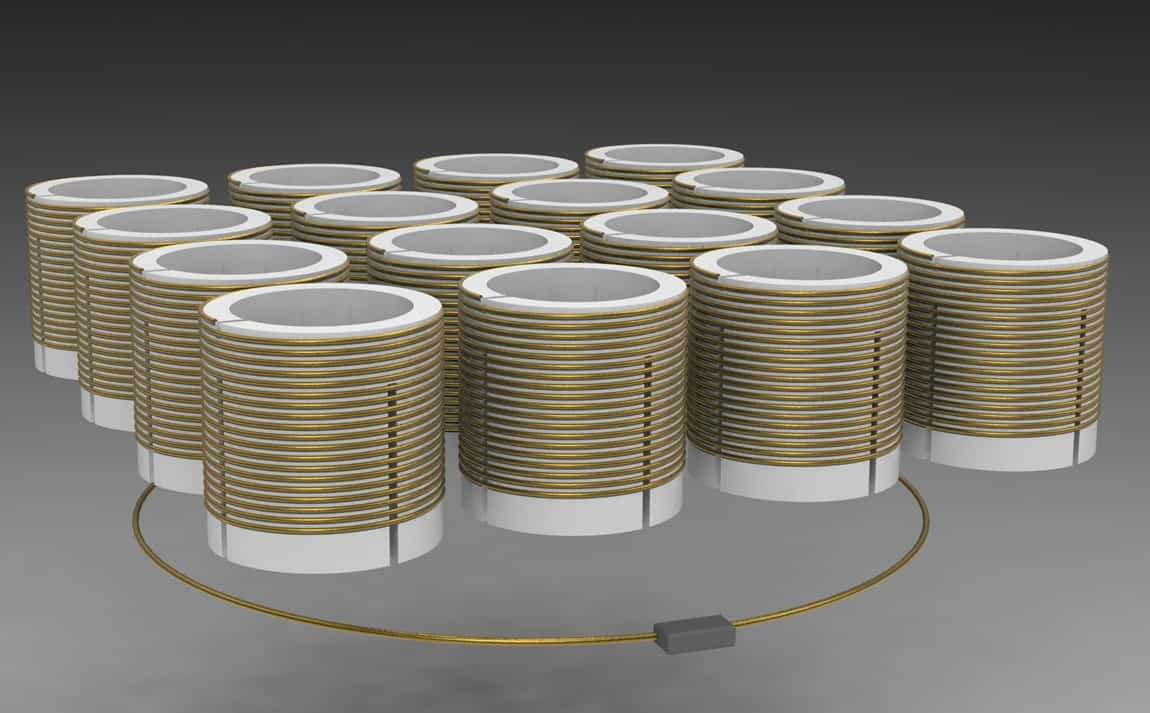
The team used models and simulations to show that the resonance frequency of the new composite material is a function of the applied electromagnetic field strength. In periods of high field strength, as is the case during proton excitation, the resonance frequency of the nonlinear metamaterial drops and switches off the amplification caused by the linear metamaterial. In contrast, when the field strength is low, as during read-out, the nonlinear metamaterial boosts the signal in the receiving coil.
“Our approach allows us to boost the received [SNR] without running into issues of excessive [RF absorption] on the transmission side,” says Zhang.
Bottles and onions
To experimentally validate the new metamaterial, the researchers imaged an oil-filled water bottle and an onion using clinical and non-clinical MR imaging sequences. They found that nonlinear metamaterials could be integrated seamlessly into a variety of clinical MR imaging protocols.
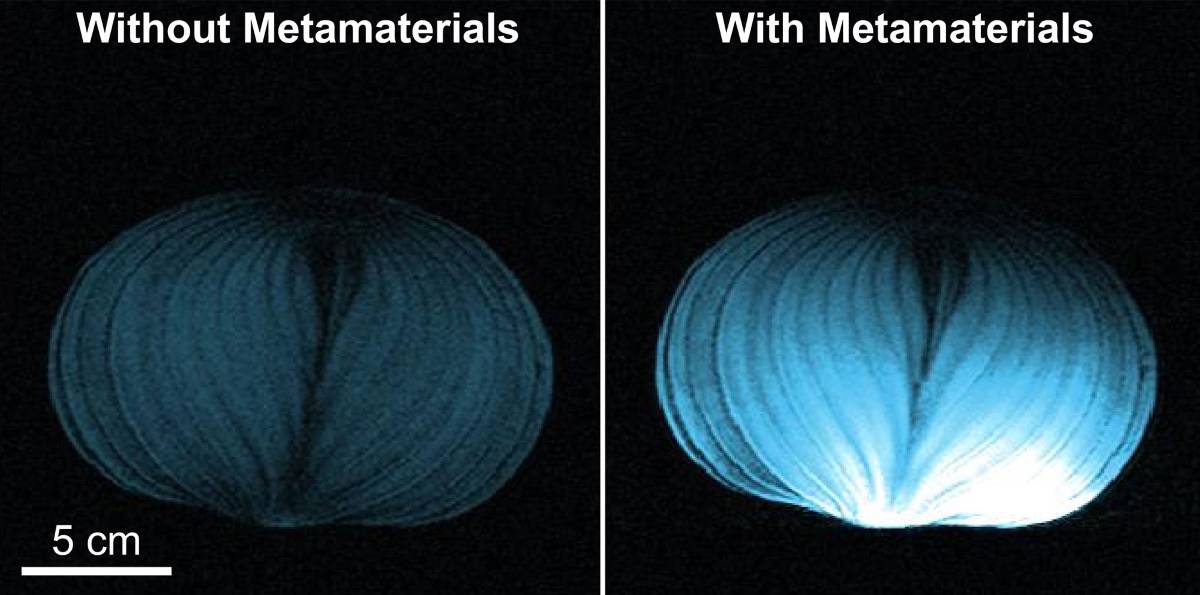
Their data revealed a near 16-fold enhancement in the peak SNR. Comparisons also showed that the SNR enhancement achieved by the nonlinear metamaterial was larger and decayed at a slower rate with increasing distance between the metamaterial and the imaging volume.
“[Nonlinear metamaterials are] several years from the clinic, [but] the approach addresses a major hurdle to clinical adoption,” Zhang maintains. Increased signal in MR imaging without increasing RF power could help reduce scan times, increase department throughput and give more patients access to the diagnostic capabilities of MR.
Not bad for a few coils of wire.