A phosphor material that emits almost twice as many photons as it absorbs has been discovered by researchers at Utrecht University in the Netherlands (R Wegh et al . 1999 Science 283 663). The discovery may lead to a new generation of highly efficient fluorescent lights and plasma displays that are also environmentally safe.
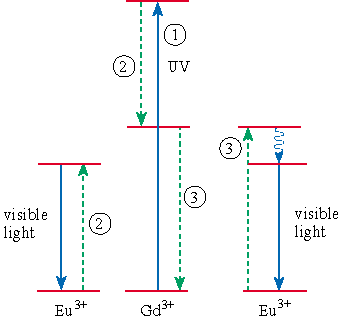
The glass tube in a conventional fluorescent light is coated on the inside with a phosphor layer and filled with a discharge material, usually mercury. Electrons excite the mercury atoms from the ground state into a short-lived excited state. Ultraviolet light is emitted as the atoms return to the ground state and this is absorbed by red, green and blue phosphors. The excited phosphor states subsequently emit the white light normally associated with fluorescent lighting. The most commonly used phosphors consist of luminescent centres – frequently lanthanide and/or transition-metal ions – incorporated into insulating inorganic oxide or halide host lattices.
The main drawback associated with fluorescent lights, however, is their reliance on mercury as a discharge material. Mercury is poisonous, making the disposal of fluorescent lights hazardous. In addition, the liquid mercury must evaporate each time the power is switched on. This warm-up period prevents the use of fluorescent lights in applications that require fast responses such as fax and photocopier machines.
These limitations have prompted a search to identify new, non-toxic discharge materials with short or no warm-up times. Among the acceptable alternatives to mercury, xenon gas is currently the most promising, as it is very efficient at producing ultraviolet light, has an immediate response and is safe. In order to create mercury-free fluorescent lights based on xenon, however, new phosphor materials must be developed. Today’s fluorescent lights are highly efficient because considerable research has been directed at identifying phosphor materials that efficiently absorb the ultraviolet light emitted by mercury. Since about 85% of the ultraviolet light produced by mercury has a wavelength of 254 nm, most of the phosphors that have been developed absorb strongly at this wavelength. White light is produced by the combined output of red, green and blue phosphors that couple efficiently to the ultraviolet output of mercury.
In contrast, most of the ultraviolet light emitted by xenon occurs at a wavelength of 172 nm, corresponding to a higher energy than that associated with mercury. As a result, new phosphors are needed that can efficiently absorb 172 nm ultraviolet light and convert it to red, green and blue wavelengths. It is relatively simple to find materials – based on the lanthanide elements, for example – that efficiently absorb the ultraviolet output of xenon. But the true challenge in working with xenon as a discharge medium is finding phosphors that efficiently convert the ultraviolet output of xenon into visible light. Two general considerations govern phosphor conversion efficiency: quantum efficiency and energy mismatch. Quantum efficiency measures the number of visible photons produced by the phosphor for every ultraviolet photon absorbed. High quantum efficiency means that once a phosphor is excited to its emitting state, it returns to its ground state primarily by emitting light. The efficiency is reduced if, instead, the excited state decays non-radiatively by dissipating heat via phonon emission. Highly efficient phosphors can be designed by varying the luminescent centre and the chemical composition of the host lattice. A quantum efficiency of close to 100% has been observed in many systems. Energy mismatch refers to the difference in energy between the photons that are absorbed and emitted by the phosphors. Since red, green and blue photons are much less energetic than ultraviolet photons, a considerable mismatch of energy is associated with most phosphors. In all phosphor materials known until now, this energy mismatch has led to non-radiative decays of the luminescent centre. Energy mismatch has therefore been an unavoidable, inefficient use of the electrical excitation energy in fluorescent lighting. The inefficiency due to energy mismatch is much more severe for xenon than mercury because of the much higher ultraviolet output energy of xenon (7.2 eV compared with 4.9 eV). The situation has changed dramatically following the Utrecht group’s discovery of the phosphor material europium-doped lithium gadolinium fluoride, LiGdF4:Eu3+. This phosphor is capable of producing red light efficiently when excited with ultraviolet light output from the xenon. The Utrecht team exploited the energy mismatch problem: since the energy of 172 nm xenon photons is more than double the energy of red, green or blue photons, it should be possible to obtain two visible photons for every ultraviolet photon absorbed by the phosphor. In other words, it should be possible to recover an extra visible photon from the energy mismatch instead of losing it non-radiatively as had always been observed previously. But the emission of two visible photons by sequential emission from a single luminescent centre had never been observed. The researchers designed a phosphor in which a luminescent centre of europium ions interacted with the lithium gadolinium fluoride host lattice. The idea was to use the gadolinium ions in the host lattice to absorb the energy from the ultraviolet light and then transfer it to europium ions to obtain visible light. If the transfer of energy occurred simply between one gadolinium ion and one europium ion, the phosphor would suffer from a significant energy-mismatch problem and would have low efficiency. However, the strategy used in the design of the LiGdF4:Eu3+ phosphor avoids this problem because two different mechanisms for transferring energy from the gadolinium ions to the europium ions are present.
The first stage involves the gadolinium ions absorbing the ultraviolet light, resulting in a highly excited state (step 1 in figure). The excited gadolinium ion next transfers energy to a europium ion (step 2). This excites the europium ion to a higher state, which subsequently emits a visible photon. During the energy transfer, the gadolinium ion decays from its original excited state to a lower-energy excited state. This excited state is still higher in energy than red, green or blue photons and the gadolinium ion continues to decay through a second energy-transfer process (step 3). This energy-transfer process returns the gadolinium ion to its ground state and excites a second europium ion to a high-energy state that decays non-radiatively to a lower-energy state, which then emits a second visible photon. Quantitative analysis of the results indicated that LiGdF4:Eu3+ has a quantum efficiency of 190%, a new record for visible light.
The Utrecht researchers have established for the first time that it is possible to obtain two visible photons from one ultraviolet photon. They used the term “quantum cutting” or “down-conversion” to describe the effect that they discovered. The process shows that fluorescent lights based on xenon discharges have the potential to be competitive with the current mercury-based lights.
Instead of being a disadvantage, the higher-energy ultraviolet output of xenon has actually proved to be advantageous. The photon produced in step 2 requires the higher-energy ultraviolet output of xenon in step 1 and would not be possible with a mercury discharge.
The discovery opens a wide range of new opportunities for xenon and other non-mercury discharges. However, the new phosphor emits primarily red light and equally efficient green and blue phosphors are still needed to make xenon-based fluorescent lighting a reality. We can expect work in this promising direction in the immediate future.