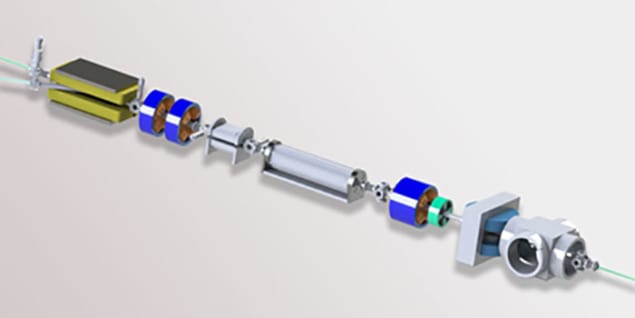
Researchers have converted the energy of an electron beam into a pulse of coherent light with an efficiency of 30% – much higher than the 0.1% efficiency of most free-electron lasers. While their demonstration produces only infrared light, the method could lead to efficient, high-power lasers operating over a range of wavelengths, including X-ray. Such X-ray sources could be used to etch circuits on semiconductor chips faster and more efficiently.
Using facilities at the Brookhaven National Laboratory in the US, the researchers demonstrated the energy conversion by first accelerating a beam of electrons in a five-metre-long tunnel. They then directed those electrons for several more metres along a helical path using magnets, before illuminating those electrons with an infrared laser.
The laser caused the electrons to slow down while emitting coherent light. Because the emitted light was the same wavelength as the laser, the process – known as stimulated emission – effectively amplified the original infrared laser beam. The degree of amplification depended on the starting energy of the electron beam, with the team saying it has broken the record for an interaction “between a free-space propagating laser pulse and a relativistic electron beam”.
The group successfully converted more than 30% of the electrons’ energy into a coherent light pulse that was three picoseconds long and had a peak power of about 100 gigawatts. Pietro Musumeci, a physicist at the University of California, Los Angeles, in the US, who was involved in the work, says that researchers first attempted this technique about 30 years ago but were only successful at converting beam energy into longer, microwave wavelengths.
Exceeding expectations
Musumeci’s group inferred the energy of the light pulse by measuring how much energy the electron beam lost and by comparing the measurements to a theoretical simulation, although the team plans to directly measure the pulse’s energy in a future experiment. He adds that it is, in principle, possible to convert up to 90% of the electron-beam energy into coherent light by making the experimental set-up longer. The laser would then stimulate the electron beam to emit for a longer time, thus converting more energy into coherent light.
The eventual goal is to create consecutive pulses to generate an efficient, high-power laser. Laser light is useful for scientific and industrial applications because it can be sharply focused and can efficiently fit “a lot of light in a small volume”, as Wim Leemans, director of the Berkeley Lab Laser Accelerator, who was not involved in the research, points out. This tightly focused light from a pulsed beam can be used, for example, to capture “snapshots” of rapid quantum phenomena.
However, coherent light sources are commonly inefficient, Leemans says. For example, an X-ray free-electron laser only has an efficiency of around 0.1%, making a technique that can convert 30% of the electron-beam energy into photon energy significant. Leemans, who is working on a portable X-ray laser that fits on a table, says that smaller laser sources could adapt this technique to achieve higher efficiencies.
Musumeci says that this technique could eventually be used to make efficient, high-power, 13 nanometre X-ray sources used to etch tiny circuits on semiconductor chips. “All the new generation chips in your iPhone and your computer are made with 13 nanometre light,” he says. “So if you have an efficient light source there, you can print more and faster.”
To tune the wavelength of the generated light pulse, he says, the infrared laser in their set-up would need to be replaced with a laser at the new wavelength, and the spacing of the magnets in the cavity would need to be changed.
The research is described in Physical Review Letters.