
Carbon nanotubes can be used as tools to more easily deliver genes into plant cell nuclei and chloroplasts, say two groups of researchers – one at the University of California at Berkeley and the other at the Massachusetts Institute of Technology (MIT). The UC Berkeley approach involves grafting DNA onto a carbon nanotube to deliver the biomolecule into plant cells while the MIT technique makes use of a mechanism called lipid exchange envelope penetration (LEEP) for delivery into chloroplasts specifically. Both techniques are very different to conventional genetic engineering methods – such as biolistics (which is done by firing genes into plant tissue) or delivering genes using infectious bacteria.
Genetically enhanced plants can be produced in higher yields and be made to be more resistant to disease and drought. This will be important for feeding a growing population in the coming decades, especially in the context of climate change. They may also be used to provide cleaner and more efficient biofuels as well as to biosynthesise pharmaceuticals.
Genetically editing plant cells is done with tools like DNA, RNA and proteins, but these biomolecules are difficult to deliver through plant cell walls, which are rigid and multi-layered structures that envelope the plant cell membrane.
Bacteria and gene guns
At the moment, the most common way to deliver genes involves making use of bacteria that naturally infect plants to deliver genes for desired traits, but this technique is efficient for only a narrow range of plant species. The DNA delivered in this way also integrates into the genome of the plant, which then means that it has to be labelled as being genetically modified (GMO). Biolistics (also known as the gene gun) is another commonly-employed technique and can “shoot” genetic material like a bullet into a wider range of plants but it is destructive and, again, inefficient. “It is like blowing a hole in a plant cell and hoping that your gene and the cell both survive,” explains Markita del Carpio Landry, who led the UC Berkeley research effort.
Landry and colleagues developed two distinct grafting methods to load green fluorescent protein (GFP)-encoding plasmids on single- and multi-walled carbon nanotubes (SWCNTs and MWCNTs) for delivery through the plant cells walls of four plants: Nicotiana benthamiana (Nb), Eruca sativa (arugula), Triticum aestivum (wheat) and Gossypium hirsutum (cotton).
High strength and needle-like aspect ratio
“Carbon nanotubes are interesting here because they are among the few nanoparticles that can be made narrow – just 1-nm-wide – which means they are small enough to slip through the plant cell wall,” she says. “Their exceptionally high strength and needle-like aspect ratio allows them to be passively internalized into the plant cells of many different species.”
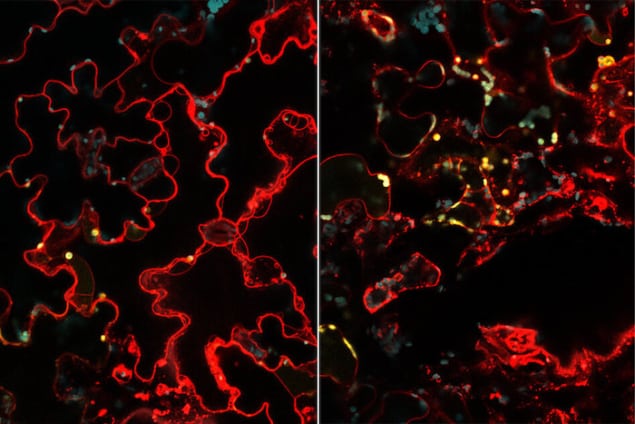
The researchers tracked the nanoparticles and found that the plants glowed green when irradiated with UV light. This shows that the GFP gene had been transcribed and translated into protein, just as it would if it was the plants’ own gene.
They also found that the CNTs end up in both the cell nuclei and in chloroplasts (which each contain about 80 of the genes that code for proteins involved in photosynthesis). Indeed, over 90% of the CNTs end up in chloroplasts
Another good thing about the nanotubes is that they act as a shield and prevent the DNA from being inserted into the plant’s genome, explains Landry. This means that the plants do not have to be designated as GMO in the US and many other countries (excluding the European Union). And that is not all: to their surprise, the researchers also found that when adsorbed onto the surface of CNTS, the DNA cargoes showed much less endonuclease-based degradation.
“Nucleases are proteins in cells responsible for degrading foreign DNA and RNA,” explains Landry. “One of the challenges for DNA and RNA delivery to many cell types, not just plant cells, is to inhibit this degradation. Our work shows that CNTs not only facilitate DNA delivery to plant cells, they may also be ‘hiding’ the DNA from being recognized and degraded by nucleases.”
Getting into chloroplasts
Meanwhile, the MIT team, led by Michael Strano has made of use of the so-called lipid exchange envelope penetration (LEEP) mechanism to deliver nanoparticles with DNA on their surface into plant cells – and more specifically into chloroplasts. Since plant cells have dozens of chloroplasts, those expressing foreign genes could allow for much larger amounts of a desired protein to be generated than is possible in only cell nuclei expressing these genes. Editing chloroplast genes in this way could help increase the amount of energy produced by photosynthesis, which, in turn would allow plants to grow bigger and more quickly, and in greater yield.
Strano and colleagues discovered LEEP a few years ago when they found that they could make nanoparticles penetrate plant cell membranes by tuning the electrical charge of the particles (so that they had a high enough zeta potential) and their size (so they were of the right dimensions).
In their new technique, the researchers wrapped single-walled carbon nanotubes in chitosan, a biopolymer that is commonly found in the shells of shrimps and other crustaceans. This chitosan-complexed-SWCNT is positively charged, which allows it to bind to negatively-charged plasmid DNA through electrostatic attraction.
Weakened binding
“We simply infiltrated this plasmid-DNA-SWCNT complex through plant leaves,” explains team member Tedrick Thomas Salim Lew. “The complex enters the leaf through stomata, passes through the cell wall and cell membrane, and finally localizes in the chloroplasts.”
Since chloroplasts are slightly more basic (pH 8) than the cytosol or the environment outside the plant cell, the chitosan-SWCNT becomes less positively charged when it reaches the chloroplasts. Binding with plasmid-DNA is thus weakened and the DNA unloads within the chloroplasts. Once unloaded it can be translated into proteins
In their experiments, the researchers delivered a gene for yellow fluorescence protein (YFP), which allowed them to easily visualize which plant cells expressed the protein. They found that roughly 47% of the plant cells did so. This figure could be increased by delivering more particles, they say.
Technique works for wide range of plant species
The new nanoparticle-mediated delivery approach is simple, easy to perform, inexpensive and works for a wide range of plant species, Lew tells Physics World. Indeed, the researchers tested it out in spinach, watercress, tobacco, arugula and Arabidopsis thaliana. “Our technique is fundamentally different to Landry and colleagues ‘since we design our nanoparticles to selectively traffic into the chloroplast and target the chloroplast genome.”
Since the chloroplast genome can only be inherited from maternal cells, the beneficial traits can be passed onto offspring, but, importantly, cannot be transferred to other, nearby, plant species,” he explains. This means that there is less risk of undesirable gene spread.
Like Landry and colleagues’ nanotubes, these nanocarriers can also protect the plasmid from enzymatic degradation.
“We believe that our nanoparticle-mediated approach is a useful complement to the plant biotechnology toolkit,” says Lew. “It could also help optimize pharmaceutical product synthesis in plant chloroplasts – some vaccines are actually produced within the chloroplasts through genetic engineering.”
Applications in crop engineering and plant biology studies could also benefit. “For example, in the future, we may be able to engineer crops that are resistant to diseases or droughts by delivering the appropriate gene to the chloroplasts.”
More permanent effects required
However, the researchers do stress that further improvements have to be made to their technique before this can happen. “The gene expression we have observed is transient (lasting only a few days), meaning that the foreign DNA is not stably integrated in the plant genome. For the offspring to inherit the genetic traits, we have to realize more permanent editing,” adds Lew.
Landry and colleagues’ technique also results in transient gene expression.
“Our studies do show, however, that we can control how nanoparticles enter plant cells with a very fine degree of precision,” Landry tells Physics World. “This will allow us to dream up future applications in transgene-free crop editing, rapid testing of how plant genes may confer desirable traits to creating more robust crops, and even engineering photosynthetic proteins that are coded in the chloroplast genome.”

Warmer springs may reduce later plant growth
“Exciting new frontier”
“We posted a bioRxiv of our CNT-based gene delivery work back in mid-2017 in the hope that our finding – that CNTs could be used to deliver genes to plants passively – would be leveraged by the scientific community. I’m now thrilled to see that these nanomaterials are being adopted for broader applications in plant transformation, such as in Strano and colleagues’ study as well as and several other research groups in the plant nanobiotechnology field, demonstrating carbon nanotube-based delivery of genes to the chloroplast.
“Plant science nanotechnology is an exciting new frontier and we are only beginning to understand how nanoparticles can squeeze through the cell wall. My lab has recently completed a study using DNA origami to look at how parameters like nanoparticle size, stiffness and even shape can affect whether or not particles make it into the plant cell. Understanding how these particles manage to passively penetrate the cell wall will help us develop better tools in the future so that we can implement other exciting tools like CRISPR for engineering the next generation of crops for an improved agriculture.”
Full details of the research from both groups are published in Nature Nanotechnology. The MIT group work is here and the UC Berkeley’s here.