Physicists in California have loaded a bunch of ultracold caesium atoms into the back of a van and driven them up a hill to demonstrate how quantum interference can be used to measure gravity outside the laboratory. When cooled to just above absolute zero, the atoms form the centrepiece of a portable gravimeter that might in future be used to measure how the Earth’s surface gradually rises to form mountains or to underpin the new physics-based definition of the kilogram.
Acceleration due to gravity varies considerably across the Earth’s surface (between about 9.78-9.83 ms-2) depending on how mass is distributed underneath. Scientists and engineers exploit these variations – in both space and time – to do things such as better understand how ice sheets melt and to monitor the build up of magma inside volcanoes.
Measuring the absolute value of gravity (g) can be done by measuring the free-fall acceleration of a corner cube reflector by bouncing laser beams off its surface and analysing the resulting interference patterns. Although very accurate, these mechanical objects are not well suited to repeated measurements in the field. Quantum gravimeters also measure objects in free-fall, but the objects in this case are atoms that experience interference effects because of their wavelike properties.
Smaller, simpler, more robust
In the latest research, Holger Müller and colleagues at the University of California, Berkeley created a portable version of such a device. As with other quantum gravimeters, it uses an atom interferometer to measure the effect of gravity on clouds of atoms that are first trapped and cooled. But its creators claim that a new kind of magneto-optical trap allows the device to be smaller, simpler and more robust than rival designs.
After being released from that trap, several million caesium atoms fall freely under gravity while being exposed to a series of laser pulses. The first pulse places the atoms in a superposition of two different trajectories through the gravitational field, while the second brings the trajectories back together. The third pulse causes the atoms to interfere and the difference in gravity along the two trajectories is revealed by the interference pattern.
Müller and team first tested their device in the lab, using it to measure Earth tides over 12 days. These miniscule distortions of the Earth, caused by the Moon’s gravity, lead to very slight oscillations in the value of g. The researchers’ results did not quite agree with model predictions and they found that the disparity was likely due to the ocean tide – which is relevant because the lab is close to San Francisco Bay. They were also able to detect the vertical acceleration of seismic waves from several distant earthquakes.
Berkeley Hills
The group then packed the interferometer, its electronics and a battery into the back of a van and drove several kilometres along a road up into the Berkeley Hills. They stopped at six points along the way and recorded how g varied with altitude – their climb of about 400 m taking them very slightly further from the centre of the Earth and therefore to a fractionally weaker gravitational field. They then compared that variation with the slightly higher gradient of g in “free air” to calculate the density of rocks in the hill.
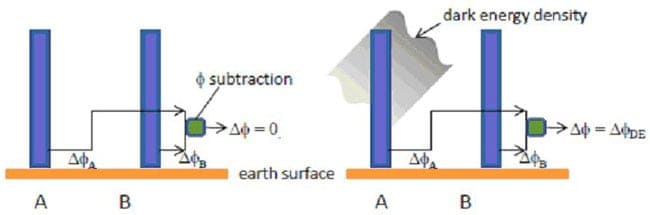
Ultracold atoms could reveal the dark sector
At each stop it took them about 15 min to power up the instrument and align the interferometer beam to the Earth’s gravitational field, and then a few more minutes to carry out the measurements. Ground vibrations limited their measurement sensitivity about 5 µm/s2. This is more than a factor of ten worse than lab-based systems, which are capable of 100 nm/s2, but Müller and colleagues claim that none have done better in the field. They say that the only other atomic instrument used to carry out gravity surveys – done on board a ship in the choppy Atlantic waters off the north-west coast of France – had a measurement uncertainty of 10 µm/s2. “With simplicity and sensitivity, our instrument paves the way for bringing atomic gravimeters to field applications,” they write in a paper uploaded to the arXiv preprint server.
Kai Bongs of University of Birmingham in the UK agrees that the new instrument’s simplicity is an important feature, arguing it could lead to quantum devices that are competitive in price with their classical counterparts (which cost about $100,000 each). But he reckons that the instrument’s sensitivity is less important. In contrast to a gradiometer, which measures relative gravity and can remove the effect of vibrations, he points out that an (absolute) gravimeter needs lots of measurements to average out vibrational noise. “It wouldn’t make sense to build an instrument a thousand times more sensitive because it would take years to reach that sensitivity limit in the real world,” he says.