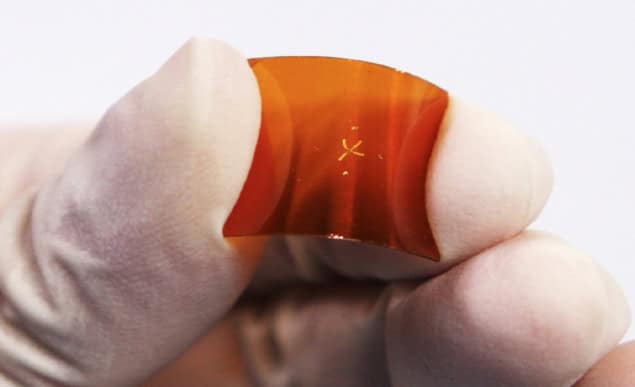
A new sensor can detect mechanical strains that are more than an order of magnitude weaker than was possible with previously reported devices. Developed at Nanjing University, China, the sensor works by detecting changes that take place in single-crystal vanadium oxide materials as they undergo a transition from a conducting to an insulating phase. The new device could have applications in electronics engineering as well as materials science.
To detect tiny deformations in materials, you ideally want a sensor that undergoes a seamless and easily measurable transition whenever a strain – even a very weak one – is applied to it. Phase transitions, such as the shift from a metal to an insulator, fit the bill because they produce a significant change in the material’s resistance, making it possible to generate large electrical signals. These signals can then be measured and used to quantify the strain that triggered them.
Traditional strain sensors, however, are based on metal and semiconductor compounds, which have resistances that don’t change much under strain. This makes it hard to detect weak strains caused by, for example, the movement of microscopic water droplets around a surface.
A research team co-led by Feng Miao and Shi-Jun Liang has now got around this problem by developing a sensor based on the bronze phase of vanadium oxide, VO2(B). The team initially chose to study this material purely to understand the mechanisms behind its temperature-induced phase transitions. Along the way, though, they noticed something unusual. “As our research progressed, we discovered that this material exhibits a unique response to strain,” Liang recalls. “This prompted us to shift the project’s focus.”
A fabrication challenge
Because the structure of vanadium oxide is not simple, fabricating a sensor from this quantum material was among the team’s biggest challenges. To make their device, the Nanjing researchers used a specially-adapted hydrogen-assisted chemical vapour deposition micro-nano fabrication process. This enabled them to produce high-quality, smooth single crystals of the material, which they characterized using a combination of electrical and spectroscopic techniques, including high-resolution transmission electron microscopy (HRTEM). They then needed to transfer this crystal from the SiO2/Si wafer on which it was grown to a flexible substrate (a smooth and insulating polyimide), which posed further experimental challenges, Liang says.
Once they had accomplished this, the researchers loaded the polyimide substrate/VO2(B) into a customized strain setup. They bonded the device to a homemade socket and induced uniaxial tensile strain in the material by vertically pushing a nanopositioner-controlled needle through it. This bends the flexible substrate and curves the upper surface of the sample.
They then measured how the current-voltage characteristics of the mechanical sensor changed as they applied strain to it. Under no strain, the channel current of the device registers 165 μA at a bias of 0.5 V, indicating that it is conducting. When the strain increases to 0.95%, however, the current drops to just 0.50 μA, suggesting a shift into an insulating state.
A strikingly large variation
The researchers also measured the response of the device to intermediate strains. As they increased the applied strain, they found that at first, the device’s resistance increased only slightly. When the uniaxial tensile strain hit a value of 0.33%, though, the resistance jumped, and afterwards it increased exponentially with applied strain. By the time they reached 0.78% strain, the resistance was more than 2600 times greater than it was in the strain-free state.

Monolayer strain sensor sets new record
This strikingly large variation is due to a strain-induced metal-insulator transition in the single-crystal VO2(B) flake, Miao explains. “As the strain increases, the entire material transitions to an insulator, resulting in a significant increase in its resistance that we can measure,” he says. This resistance change is durable, he adds, and can be measured with the same precision even after 700 cycles, proving that the technique is reliable.
Detecting airflows and vibrations
To test their device, the Nanjing University team used it to sense the slight mechanical deformation caused by placing a micron-sized piece of plastic on it. As well as detecting the slight mechanical pressure of small objects like this, they found that the device can also monitor gentle airflows and sense tiny vibrations such as those produced when tiny water droplets (about 9 μL in volume) move on flexible substrates.
“Our work shows that quantum materials like vanadium oxide show much potential for strain detection applications,” Miao tells Physics World. “This may motivate researchers in materials science and electronic engineering to study such compounds in this context.”
This work, which is detailed in Chinese Physics Letters, was a proof-of-concept validation, Liang adds. Future studies will involve growing large-area samples and exploring how to integrate them into flexible devices. “These will allow us to make ultra-sensitive quantum material sensing chips,” he says.