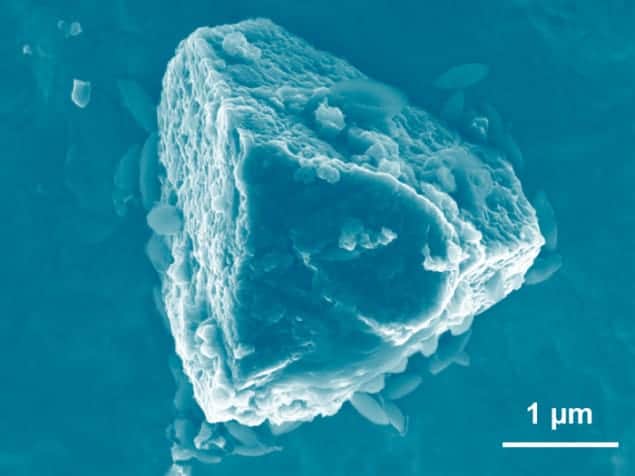
A newly discovered state of the sulphur-31 nucleus could help to explain the puzzling isotope ratios found in tiny grains of silicon carbide that are found in some meteorites. The discovery was made at the National Superconducting Cyclotron Laboratory in the US, and provides important information about how elements such as silicon are created in stellar explosions called novae.
Silicon is the eighth most common element in the universe by mass and it comprises nearly 30% of the Earth’s crust. There are three stable isotopes of silicon: 3% of the silicon on Earth is silicon-30, 5% is silicon-29 and the remainder is silicon-28. In 2001 researchers in the US and Spain found five microscopic grains of silicon carbide in a meteorite that contained up to twice as much silicon-30 as found in rocks on Earth. The team suggested that the isotopic anomaly occurs because the grains had been created in classical novae – huge thermonuclear explosions that occur on the surfaces of stars and produce heavy elements in a process called nucleosynthesis. Grains subsequently found in other meteorites have also been attributed to classical novae.
The idea is that phosphorus-30 nuclei created in a nova will decay to silicon-30. For this to happen, however, the phosphorous-30 nucleus must avoid capturing a proton before it decays – because this would result in the formation of sulphur-31. The rate at which this proton capture occurs increases with increasing temperature, and therefore the hotter the nova, the fewer silicon-30 nuclei it produces. The problem with this theory is that calculations based on what we know about nova nucleosynthesis suggest that even more silicon-30 should be present in nova dust grains than has been measured. This has led some researchers to ask whether the grains have another origin.
Challenging cross-section
A crucial input parameter for these calculations is the probability that phosphorous-30 will capture a proton at temperatures found in novae. Called the proton capture cross-section, this has so far proved impossible to measure experimentally, explains team-member Christopher Wrede of Michigan State University (MSU). “You can’t make a target out of phosphorus-30 and bombard a proton beam onto the target,” he says. “Instead, you have to make a target out of protons – hydrogen – and bombard that with a beam of phosphorus-30. It’s very challenging to make a sufficiently intense beam.” As a result, astrophysicists have had to use rough theoretical estimates in their calculations of silicon-30 production.
Wrede and colleagues took a different approach based on the fact that the cross-section depends on the precise energies of unbound sulphur-31 nuclear states (called resonances) that are formed when phosphorus-30 captures a proton. Conveniently, these resonances are the same as those formed when the rare isotope chlorine-31 undergoes beta decay to sulphur-31. The team studied the decay of chlorine-31 ions produced at MSU’s National Superconducting Cyclotron Laboratory, measuring the beta particles and subsequent gamma rays that are emitted.
Identical resonance
The researchers found a new sulphur-31 resonance with energy, spin and parity identical to the resonance formed when phosphorus-30 captures a proton. This, says Wrede, suggests that the capture cross-section is larger than previous estimates. This means that more phosphorus-30 nuclei are converted to sulphur-31 in novae, resulting in fewer silicon-30 nuclei. While this finding goes some way to explain the isotopic composition of the meteorite grains, “we really need to know how strong this resonance is before we can verify that”, Wrede says.
As David Jenkins of the University of York in the UK comments, “What’s surprising is that, despite the very large number of studies of this system, somebody has found something new that wasn’t known before. Previous estimates [of the proton capture cross-section] that have gone into the astrophysical models are clearly inaccurate and incomplete.” He adds, however, that the research still does not pin down the rate at which sulphur-31 is produced in novae. “The obvious further work, which a lot of people have been trying to do, is to try and measure this phosphorus-30 reaction rate directly,” he says.
Wrede and colleagues are hoping to do just that. “There’s a facility being built at out lab called Separator for Capture Reactions (SECAR),” says Wrede. “We hope that in 5–7 years, it will be capable of measuring the proton capture into this resonance directly on phosphorus-30.” The chlorine-31 result will assist this endeavour, he says, because “we will have to tune the beam energy to the exact resonance energy to make the measurement efficiently.” Measuring this cross-section could not only solve the mystery of the grains, the researchers say, but also help astronomers, who often estimate the temperature of novae from the proportion of elements heavier than phosphorus.
The research is described in Physical Review Letters.