
In vivo imaging plays an essential role within medicine, pharmaceutics and biology. Imaging with fluorescent tracers is one promising approach, as multiple tracer types are available to simultaneously visualize the distribution of numerous molecules. Biological tissues, however, will scatter and attenuate visible light, making image reconstruction and quantitative measurements of fluorescent dyes difficult.
Instead, researchers can employ X-ray-emitting radionuclides for in vivo imaging, using techniques such as single photon emission computed tomography (SPECT) and positron emission tomography (PET) to image tracers deep inside the body. Multi-radionuclide imaging, however, is tricky. There are far fewer radionuclides available for imaging compared with the number of fluorescence dyes, and the energy ranges of some of their emission lines can be similar, or even overlap.
What’s needed is a high-sensitivity detector, with high spatial resolution and good enough energy resolution to resolve potential overlaps between multiple radiotracers. To achieve this, biomedical researchers in Japan have teamed up with their colleagues in astronomy to adapt technology used in space observations for biomedical research.
Led by Atsushi Yagishita, from Kavli IPMU and the National Cancer Center Japan, the researchers developed the IPMU imager – a biomedical imaging system based on a cadmium telluride detector originally developed for hard X-ray and gamma-ray space observations. The imager exhibited high spatial and energy resolution, but could not completely eliminate all noise, especially when imaging radionuclides with similar emission lines. So the researchers improved it further by employing a spectral analysis method used in X-ray astronomy.
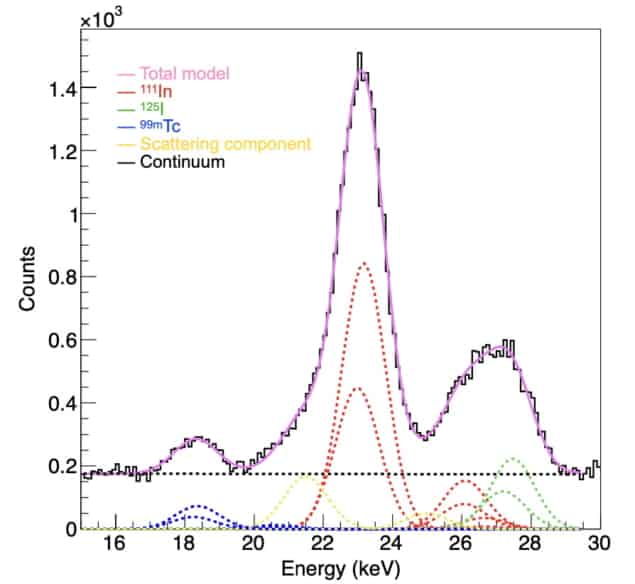
When imaging multiple radionuclides, the goal is to generate spectra and images from individual radionuclides, without contamination from other emitters in the sample. The spectroscopic analysis method involves fitting the observed spectra to a model of radionuclide emission lines in specific energy bands. Then, using the determined intensity ratios between the nuclides, any contamination in the image can be calculated and eliminated. The researchers, also from RIKEN and the Institute of Space and Astronautical Science/JAXA, describe the new imaging technique in Nature Biomedical Engineering.
System tests
To verify their new approach, the researchers measured solutions of the gamma-ray emitters iodine-125 (125I) and indium-111(111In). They examined a pure 125I sample, and a sample with the same activity of 125I mixed with 111In. Their analysis method completely separated spectra from the individual radionuclides and the background component. After processing, 125I images from both samples exhibited the same signal intensity, demonstrating the ability to remove noise from other radiation sources and obtain accurate images of only the target radionuclide.
“We assessed the quantitative performance of the images when using multiple radionuclides and confirmed that the intensity of the images correctly represented the radioactivity,” Yagishita tells Physics World.
The researchers also evaluated the spatial resolution of the IPMU imager, by imaging a phantom containing various sized holes filled with solutions of 111In, 125I or technetium-99m (99mTc) and reconstructing images in the 21–25, 26–29 and 135–143 keV energy bands, respectively. For all samples, the images clearly showed the 350 and 500 μm holes, while some of the 250 μm holes were also distinguished. The team note that this spatial resolution of roughly 300 μm is similar to that of state-of-the-art small-animal SPECT systems.
In vivo experiments
Finally, Yagishita and colleagues used the IPMU imager to simultaneously visualize thyroid tissue, mandibular lymph nodes and parotid lymph nodes in mice, using 125I, 111In and 99mTc, respectively. The spectrum obtained with the IPMU imager clearly showed the separate emission lines from the three radionuclides.
The team then reconstructed images of the three tracers in the relevant energy bands. While the raw images contained noise and ghosting caused by emission from the other radionuclides, the fitting technique identified unwanted radiation sources and produced separate images of each radionuclide in vivo.
In images of 125I (in the 26–29 keV energy band), the thyroid gland where iodine accumulates could be accurately delineated by subtracting background noise and 111In contamination. The researchers note that the processed image showed no activity in the lymph nodes, where 125I does not accumulate.

Compton camera simultaneously images PET and SPECT tracers in human body
For comparison, they imaged the same mouse using a conventional small-animal SPECT/CT scanner. The low-energy-resolution spectrum only showed a single peak, while SPECT images in the 125I energy window showed the lymph nodes more clearly than the thyroid. These results suggest that the IPMU image is of higher quality than the SPECT image.
In future studies, the team hopes to use the IPMU imager to visualize targeted radionuclide therapy (TRNT) drugs in vivo. “TRNT drugs target specific landmarks on cancer cells and accumulate in cancer cells,” Yagishita explains. “Although the drug pharmacokinetics is ordinarily invisible, it is theoretically possible to visualize TRNT drugs because some emit gamma rays or X-rays. So currently, we are focusing on visualizing the pharmacokinetics of TRNT drugs.”