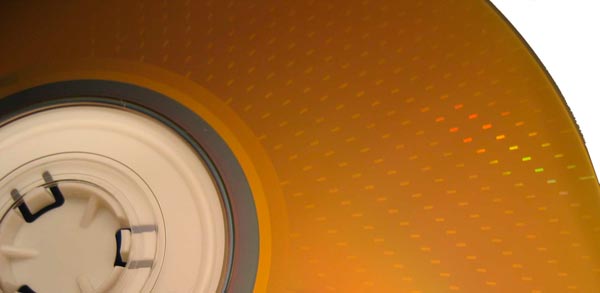
A new microscopy technique has allowed researchers in the US to make the first measurements of the earliest stages of crystallization. The technique could help scientists to gain a more complete understanding of how materials crystallize – which might eventually lead to high-speed computer memories based on crystallization.
Crystallization usually starts with the formation of nanometre-sized crystalline clumps called nuclei, which increase in size to take over the entire material. The classical theory of nucleation assumes that the tiny clumps are constantly forming and breaking apart, and it is only when they reach a critical size that they can trigger runaway crystallization.
Although the presence of these “sub-critical” nuclei had been predicted decades ago, it has been extremely difficult to observe how they evolve and affect crystallization, according to Bong-Sub Lee, a materials scientist at the University of Illinois at Urbana-Champaign, who played a leading role in this latest research.
Using fluctuation
To address these problems, the team used a modified form of transmission electron microscopy (TEM) called fluctuation TEM. This technique involves recording hundreds of electron diffraction patterns from a single sample, and then using statistics to build up a histogram showing the number of nuclei observed as a function of size.
According to Lee, an important advantage of this method over other techniques is that it can easily pick out crystalline regions 1–2 nm in size from the background noise. A conventional high-resolution TEM is limited to nuclei larger than about 4 nm
The team studied an alloy of antimony, indium, silver and tellurium called AIST, which is used to make rewritable CDs and DVDs. By heating the alloy with a laser, regions of the AIST can be switched back and forth between crystalline and amorphous forms, allowing binary data to be encoded on the disk. Heating to 250–400 °C produces the crystalline phase, whereas heating to 600 °C followed by rapid cooling produces the amorphous phase.
Super-criticality takes time
By combining fluctuation TEM with a pulsed-laser technique, the team watched these processes in action. “We observed that if a particle becomes bigger than a critical size it can keep growing to become a big crystal. But it takes time to make such a super-critical nucleus. If you have a lot of smaller sub-critical nuclei already embedded in the material, this waiting can be shortened,” Lee told physicsworld.com.
Lee’s team was able to show that it is possible to increase the number of embedded nuclei in the material – priming it for crystallization. This was done by annealing the material – heating it up and letting it cool slowly.
Their results agree with numerical simulations based on the classical theory and showed that even if two amorphous materials have the same composition, the crystallization speed may be vastly different depending on how they are prepared.
Memory potential
AIST and other “phase change” materials are currently being investigated for use in miniaturized memory chips for use in computers or mobile phones. “This technique gives new insight into the initial formation mechanisms of crystalline nuclei, so it may prove very helpful,” says Matthias Wuttig at Aachen University in Germany.
“Phase change materials have a number of exciting scientific characteristics that make them promising,” Wuttig explains. “They can very rapidly switch between amorphous and crystalline states, and there is a pronounced contrast in properties between the two.”
Lee’s team has already started looking at other materials – and because nucleation and crystallization are key processes in nature, they hope to extend the work to experimentally test other long-standing theoretical predictions in nucleation science.
The work is described in Science.