
FLASH radiotherapy, in which therapeutic radiation is delivered at a very high dose rate, shows promise for sparing normal tissues while maintaining the tumour kill seen in conventional radiotherapy. Most studies to date have been performed using electron beams, but FLASH irradiation could also be delivered using protons.
Proton therapy delivers a low integral dose to normal tissue and spares tissue behind the tumour, courtesy of the Bragg peak – the depth at which the proton beam deposits the majority of its dose. But this precision targeting brings its own problems: margins are needed to account for inexact Bragg peak positioning; robust planning is required to mitigate organ motion and anatomical changes; and uncertainties surround the distribution of linear energy transfer (LET) and relative biological effectiveness (RBE) near the Bragg peak.
So could combining protons with FLASH delivery eliminate these shortcomings? According to Frank Verhaegen from Maastro Clinic, it may actually enable a completely new way of delivering protons, one that doesn’t rely on the Bragg peak at all. “A while ago, I had the idea that if we could do FLASH protons, we could get rid of the ‘tyranny’ of the Bragg peak,” says Verhaegen.
The idea is that instead of positioning Bragg peaks inside the target, the proton beams have sufficient energy to travel straight through and exit the patient. This approach effectively positions the Bragg peaks distally in air, a tactic that’s already common in preclinical research where precision targeting is difficult inside small animals.
Instead of relying on the Bragg peak for tissue sparing, this shoot-through technique exploits the protective effect of FLASH irradiation on healthy tissues. “With FLASH, normal tissue is spared not because of the low dose, but exactly because it is normal tissue, which responds to ultrahigh dose rates in an entirely different fashion,” Verhaegen explains. “Then you can just shoot protons through the patient, and even use them behind the patient to perform proton portal imaging.”
Verhaegen and colleagues have now examined the use of shoot-through FLASH proton therapy in an illustrative brain tumour case, reporting their findings in Physics in Medicine & Biology.
Proof-of-principle
The researchers created a proof-of-concept proton plan for a patient with a neurological tumour close to several organs-at-risk (OAR) with strict dose constraints. The plan used four proton beams aimed at four fictitious targets placed outside the patient, with the beams optimized to deliver a roughly uniform dose inside the target. The team then compared this fictitious shoot-through FLASH plan with a conventional clinical four-beam proton plan.
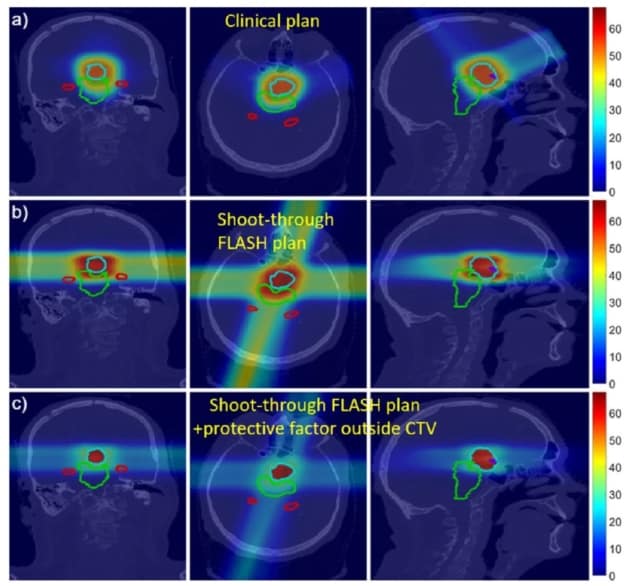
For the shoot-through plan, the researchers assumed a hypothetical FLASH protective factor for normal tissues of 2. They note that the sparing effect observed to date in electron-based FLASH studies lies between 1.4 and 1.8. Higher protective factors have been reported for FLASH proton beams, though it is unknown if or why FLASH protons would exhibit greater protection.
Preliminary dose calculations showed that the shoot-through plans delivered an acceptable dose to the target. In most cases, the OAR dose constraints were met or almost met. And for some OARs, the FLASH effect offers potential to lower the effective dose below the planning constraints. The shoot-through beams increased the integral dose to the brain, but incorporating the FLASH protective factor of 2 reduced the effective dose to near that of the non-FLASH clinical plan.
The team points out that the treatment planning system used in this illustration was not optimized for shoot-through FLASH, and that an algorithm developed specifically for this novel modality should meet OAR constraints more easily.
Looking ahead
Clinical implementation of shoot-through FLASH proton therapy could provide a range of benefits, including the ability to perform dosimetry via proton portal imaging. Up to now, such verification of proton therapy has been elusive, although there is a great need for it. The shoot-through approach would also mostly remove the need for treatment margins and eliminate the problem of LET and RBE uncertainties.
Importantly, accelerators that can deliver shoot-through treatments for tumours in the head, neck and thorax, using 230–250 MeV proton beams, already exist. Abdominal treatments would need higher proton energies (300–350 MeV), though this would not require new accelerator technology, only an upscaling. Accelerators could also be considerably simpler than current implementations, for example, by removing the need for beam energy modulators.
The team notes that laser-based proton accelerators could offer a big advantage for FLASH because of their ultrahigh dose rate, but may require still significant development to reach high enough energies for shoot-through treatments.
Ultimately, the clinical impact of shoot-through FLASH proton therapy will depend heavily upon the FLASH protective factor, which remains an unknown quantity. “The protective factor will possibly depend on a lot of parameters, some of which may be unknown at this moment since we don’t have an explanation yet for the FLASH effect,” Verhaegen noted.
Following this proof-of-principle study, the team is now studying further neurological cases, including different sized tumours located close to sensitive structures, Verhaegen tells Physics World.