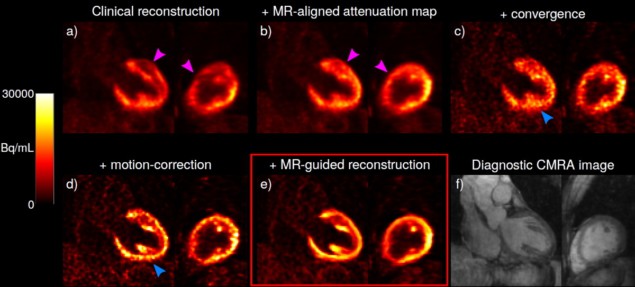
Cardiac positron emission tomography (PET) with the radiotracer 18F-FDG is used to measure the health of the heart muscle, and can detect tissue damage and scarring following a heart attack. The quality of the PET images, however, can be impaired by respiratory and cardiac motion, reducing their diagnostic accuracy. A study headed up at King’s College London has shown that simultaneous PET–MR scanning combined with a single image reconstruction framework enables the comprehensive assessment of cardiovascular disease with improved PET image quality.
The benefit is twofold: MR-derived respiratory information enables motion correction, while MR-based anatomically guided image reconstruction suppresses noise and increases contrast in the images. This latter technique, which had previously only been demonstrated in brain PET (which doesn’t suffer from physiological motion), significantly improved PET image quality compared with alternative reconstruction methods, as the research team reports in the Journal of Nuclear Medicine.
Cardiac PET with 18F-FDG is usually performed to monitor the heart in patients diagnosed with coronary artery disease, to determine the extent of infarcted areas and evaluate myocardial damage produced by previous coronary blockages. The exam also helps to determine whether coronary stenting or bypass surgery is more appropriate for a patient.
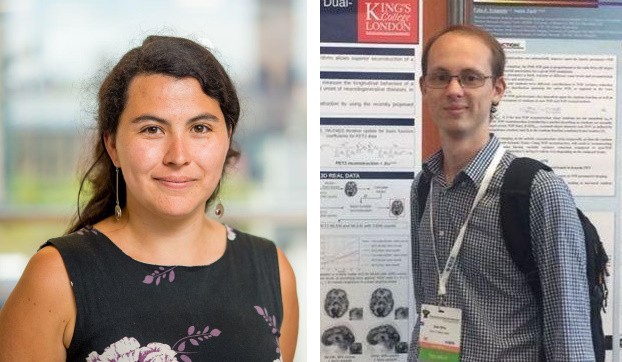
Co-principal investigators Camila Munoz and Sam Ellis and colleagues developed a single cardiac PET–MR image reconstruction framework, which uses MR-derived information to allow both motion compensation and anatomical guidance within the reconstruction of cardiac PET images. The framework resolves previous image degradation issues, such as artefacts, blurring and noise, by utilizing MR-based attenuation map alignment, respiratory motion correction, cardiac gating and MR-guidance image reconstruction.
Images are acquired using a cardiac imaging protocol designed for simultaneous diagnostic PET and coronary MR angiography (CMRA). The framework improves myocardial PET image reconstruction by aligning attenuation maps to the end-expiration respiratory position, using the CMRA images as reference to reduce attenuation-induced artefacts. It also incorporates MR-derived motion information into a motion-corrected PET image reconstruction, and uses the high-contrast, motion-corrected 3D CMRA images for anatomically guided PET image reconstruction, suppressing noise while preserving quantification performance.
Clinical assessment
To evaluate their framework, the researchers imaged five cancer patients without known or suspected cardiovascular disease. This enabled them to quantify the effect of each of the aforementioned improvements upon the final image quality. They then used the same protocol to image 10 patients with symptomatic coronary artery disease, chronic total occlusion of a relevant coronary artery and evidence of wall motion abnormalities.
Munoz, Ellis and colleagues applied a variety of image reconstruction techniques to the PET datasets and assessed the resulting image contrast and noise. They measured contrast as the contrast recovery coefficient between the left-ventricular myocardium and blood pool, and calculated noise as the standard deviation of voxels within the myocardium.
After comparing various established PET image reconstruction approaches for the two patient groups, the researchers report that each of the approaches incrementally increased contrast in the images, although this was usually at the cost of increased noise. However, their proposed framework incorporating MR-guidance further improved contrast while reducing noise. Notably, the myocardium-to-blood pool contrast increased by 143% on average compared with conventional uncorrected, non-guided PET images. Anatomical guidance reduced image noise by 16.1% compared with non-guided reconstruction.
While these findings are promising, further improvements might be needed to reduce noise to a clinically acceptable level. The researchers suggest that this can be achieved with better MR-based guidance information or by modelling the uncertainties of guidance information.
“Our results suggest that using MR information to correct for patients’ physiological motion and perform patient-specific denoising may allow for a more accurate reconstruction of the underlying distribution of PET radiotracer, in our case, 18F-FDG. This could give clinicians confidence that the cardiac PET images they are reading are a faithful representation of patients’ physiology, without artefacts from motion, image noise or other reconstruction artefacts,” explain Munoz and Ellis.

Deep learning enables safer heart scans with lower radiotracer dose
“In particular, in our results, we observed an improved depiction of myocardial viability defects, represented as an increased contrast-to-noise ratio between defect and surrounding healthy tissue,” they add. “We think that this could result in an increased diagnostic sensitivity to myocardial defects in clinical practice, by enabling the detection of smaller defects and improving delineation of the extent of the diseased tissue. However, further research is required to study the impact of these improvements in clinical decision making.”
Munoz and Ellis also point out that novel radiotracers besides 18F-FDG have recently been introduced for myocardial PET–MR imaging. “Some of these, such as 18F-NaF, generally produce very poor-quality PET images due to both physiological motion and the low radiotracer uptake from the biological processes in question,” they explain. “We believe that our motion-corrected, MR-guided PET reconstruction could prove especially useful in these situations, aiding in bringing these state-of-the-art radiotracers into clinical practice.”