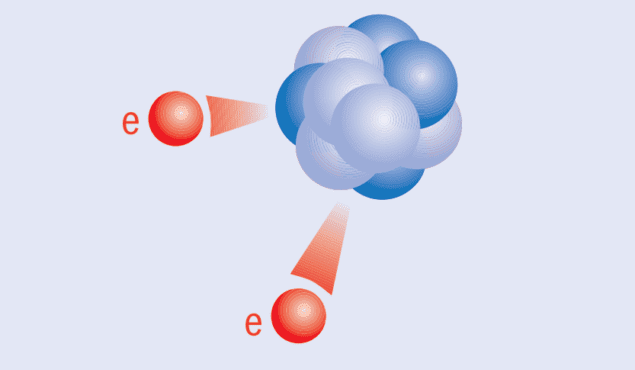
A new technique to enable the detection of a hypothetical process called neutrinoless double beta decay has been developed by an international team of physicists. Their technique involves probing a large sample of xenon for nuclei created by the decay process. If neutrinoless double beta decay is indeed spotted, it could have profound consequences for our understanding of the universe.
One of the central questions in particle physics is whether neutrinos are their own antiparticles. If they are, neutrinoless double beta decay – which is forbidden by the Standard Model of particle physics – should be possible. Several experiments are therefore competing to detect or rule out this exotic decay, which is predicted to be extremely rare.
Beta decay covers a family of processes that involve the emission of neutrinos (or antineutrinos) by a nucleus. One common beta-decay process involves a neutron in a nucleus transforming to a proton by the emission of an electron and an electron antineutrino. If neutrinos are there own antiparticles, the emitted electron antineutrino can then be absorbed as a neutrino by another neutron in the nucleus – leading to a second beta-decay and emission of another electron. While there is no overall emission of neutrinos, the nucleus has undergone a double beta decay and is left with two additional protons.
Explaining neutrino mass
Neutrinos being their own antiparticles could help explain why neutrinos have mass – something that is not explained by the Standard Model of particle physics. “We have to find mechanisms to generate neutrino mass,” explains theoretical particle physicist Werner Rodejohann of the Max Planck Institute for Nuclear Physics in Heidelberg: “The vast majority of these mechanisms predict neutrinos to be their own antiparticles.”
Rodejohann continues, “In the Standard Model without neutrino mass, you would have the same amount of matter and antimatter produced in the early Universe. These would then have annihilated leaving a Universe with only radiation. But since we are here, we know that something must have allowed a small asymmetry between matter and antimatter. Seeing neutrinoless double beta decay would confirm a lot of our ideas about how something survived.” Observing this, however, is extremely difficult.
The Enriched Xenon Observatory (EXO) in New Mexico looks for neutrinoless double beta decay in 200 kg of liquid xenon enriched in xenon-136, which is a neutron-rich nucleus that is considered a prime candidate for the process. The idea is that the two high-energy electrons emitted in the decay process ionize other xenon atoms, producing an electron shower that can be detected.
Search for barium
One challenge facing EXO physicists is how to distinguish these exceedingly rare events from other types of radioactive decay that can also produce a shower of electrons. The solution, according to William Fairbank of Colorado State University and colleagues, is to look for the barium-136 nucleus that is produced by the double beta decay of xenon-136. “None of the other likely background decays would produce a barium-136 atom at the decay site,” he explains.
Fairbank and colleagues have therefore developed a technique to retrieve single barium ions from a tank of liquid xenon using a cryogenic solid xenon probe with a small sapphire window on the end. They showed that barium ions can then be identified using laser spectroscopy. The researchers suggest that further development of the technique may provide a means to suppress the background signal in their next experiment – which will be called nEXO and will contain five tonnes of enriched xenon.
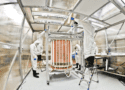
Exotic nuclear decay observed in dark-matter detector
Another challenge facing the researchers is that xenon-136 in known to undergo conventional double beta decay. This is an extremely rare process that produces two electrons and two antineutrinos. Identification of a barium ion would prove only that double beta decay had taken place, not that it was neutrinoless. To further discriminate between the two processes, the energy of the emitted electrons must also be measured to work out if some energy has been taken away by the two neutrinos. The researchers calculate that, in the energy range of interest, there is about a 50% chance of detecting a two-neutrino double beta decay would be expected in the ten years that nEXO is expected to run.
Particle physicist Ben Jones of the University of Texas at Arlington — one of the leaders of the NEXT (Neutrino Experiment with Xenon TPC) collaboration – which searches for neutrinoless double beta decay using high pressure xenon gas, describes the nEXO team’s ability to detect barium atoms as “a very substantial advance”. He adds, “So far every experiment to detect this decay has been limited by backgrounds from other radioactivity. The detection of single barium ions could reduce backgrounds to a negligible level, which would be really paradigm shifting for the field.” However, Jones cautions that there is still much more work to be done before the technique is viable.
The research is described in Nature.