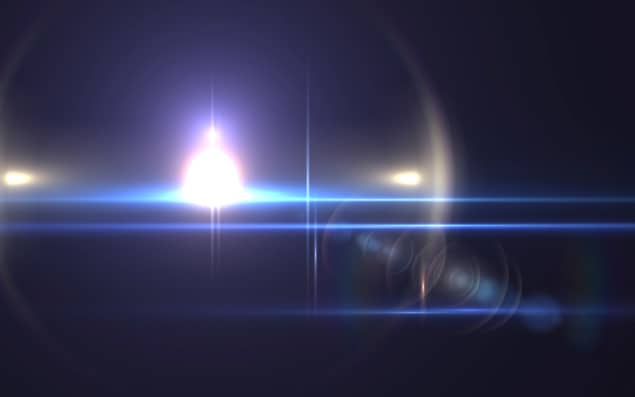
A new trick that enables photons to interact with one another has been developed by physicists in Canada. Using an ultracold gas of rubidium atoms, the researchers have shown that a single photon can have a measurable effect on the state of a separate photon beam. They say that the result marks an important step on the road to developing quantum computers that encode information using light, rather than matter.
In many ways, photons are the ideal data carrier for quantum information systems, but, given their lack of charge, they do not interact with each other. This is a particular problem in the development of quantum computers – the logic gates used in classical or quantum computers require the entities that encode bits to interact with one another.
Photonic interplay
To get round this problem, matter could be used as an intermediary – a material’s atomic state could be altered by one photon, and that in turn would change the state of a second photon. But this effect is very weak – only since the development of the laser in 1960 has it been possible to produce high-intensity light beams that have a measurable effect on each other. Unfortunately, quantum technology requires that data be encoded in individual photons, rather than in beams.
In the latest work, Aephraim Steinberg and colleagues at the University of Toronto have shown how a single photon – a “signal” beam – fired into a gas of rubidium-85 atoms cooled down to a few micro-kelvins can alter the state of a “probe” beam that travels through the gas in the opposite direction. To do so, they tune the frequency of the probe to equal that of one of rubidium’s principal transitions. They then use a third laser to etch out a very narrow “transparency window” within the absorption line so that the probe can travel unimpeded through the gas. The job of the signal photons is then to very slightly modify the rubidium’s resonant frequency, which allows the probe photons to be momentarily absorbed and re-emitted, so changing the probe’s phase and delaying it slightly.
Clicks and shifts
While the interaction of a single photon with a beam has been studied in the past, an actual single “signal” photon was never used experimentally – it was later corrected for in calculations. While Steinberg and colleagues also do not generate true single-photon beams, they reduce the intensity of the signal to the point where it likely contains either one or zero photons, and use a detector that “clicks” only if the signal contains a photon. By continuously measuring the phase of the probe after it has crossed the gas, they can establish whether or not the clicks and phase shifts go hand in hand.
Repeating this process many times, the researchers did observe such a correspondence – they found that the single photons, on average, rotate the phase of the probe beam by about one thousandth of a degree. “This dependence arises because before we measure the signal, we have entanglement between that beam, with an uncertain photon number, and the probe beam, which thereby picked up an uncertain phase,” says Steinberg.
Going from this result to a working all-optical quantum logic gate will require much additional work, however. For one thing, the effect needs to be much bigger. According to Steinberg, a phase shift of a few degrees per single photon might be enough for a working computer. While the team’s existing technique probably won’t allow that, increasing the phase shift by upping the density of the rubidium atoms may help.
Practical considerations
Another challenge will be generating interactions between two sets of single photons, rather than an individual photon and a beam of photons. The solution there, says Steinberg, might be to use the probe beam as a “quantum bus” (used to store or transfer information between independent qubits), which can interact with multiple signal beams and thereby set up connections.
Steinberg acknowledges that physicists have long been trying to generate phase shifts of 180°. A rival technique may succeed – it involves firing pairs of photons into a cloud of rubidium atoms such that the energy of one of the photons is shared with several of the atoms, and this “Rydberg state” then changes the gas’s refractive index for the other. But he points out that, in 2006, Jeffrey Shapiro at the Massachusetts Institute of Technology calculated that such a large phase change would introduce enough noise into the photon–atom system to destroy the delicate quantum state. “It remains an open question as to whether one can exploit loopholes in his theorem,” says Steinberg.
The research is published in Nature Physics.