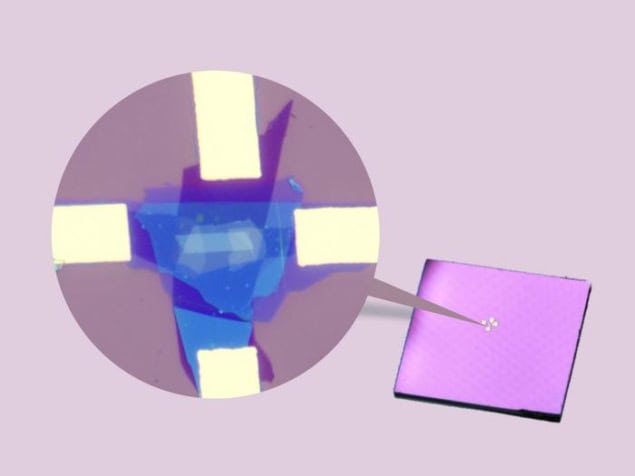
Three years ago, researchers from institutions in the US and Israel discovered a new type of ferroelectricity in a material called boron nitride (BN). The team called this new mechanism “slidetronics” because the change in the material’s electrical properties occurs when adjacent atomically-thin layers of the material slide across each other.
Two independent teams have now made further contributions to the slidetronics field. In the first, members of the original US-Israel group fabricated ferroelectric devices from BN that can operate at room temperature and function at gigahertz frequencies. Crucially, they found that the material can endure many “on-off” switching cycles without losing its ferroelectric properties – an important property for a future non-volatile computer memory. Meanwhile, a second team based in China found that a different sliding ferroelectric material, bilayer molybdenum disulphide (MoS2), is also robust against this type of fatigue.
The term “ferroelectricity” refers to a material’s ability to change its electrical properties in response to an applied electric field. It was discovered over a 100 years ago in certain naturally-occurring crystals and is now exploited in a range of technologies, including digital information storage, sensing, optoelectronics and neuromorphic computing.
Being able to switch a material’s electrical polarization over small areas, or domains, is a key part of modern computational technologies that store and retrieve large volumes of information. Indeed, the dimensions of individually polarizable domains (that is, regions with a fixed polarization) within the silicon-based devices commonly used for information storage have fallen sharply in recent years, from roughly 100 nm to mere atoms across. The problem is that as the number of polarization switching cycles increases, an effect known as fatigue occurs in these conventional ferroelectric materials. This fatigue degrades the performance of devices and can even cause them to fail, limiting the technology’s applications.
Alternatives to silicon
To overcome this problem, researchers have been studying the possibility of replacing silicon with two-dimensional materials such as hexagonal boron nitride (h-BN) and transition metal dichalcogenides (TMDs). These materials are made up of stacked layers held together by weak van der Waals interactions, and they can be as little as one atom thick, yet they remain crystalline, with a well-defined lattice and symmetry.
In one of the new works, researchers led by Kenji Yasuda of the School of Applied and Engineering Physics at Cornell University made a ferroelectric field-effect transistor (FeFET) based on sliding ferroelectricity in BN. They did this by sandwiching a monolayer of graphene between top and bottom layers of bulk BN, which behaves like a dielectric rather than a ferroelectric. They then inserted a parallel layer of stacked bilayer BN – the sliding ferroelectric – into this structure.
Yasuda and colleagues measured the endurance of ferroelectric switching in their device by repeatedly applying 100-nanosecond-long 3V pulses for up to 104 switching cycles. They then applied another square-shaped pulse with the same duration and a frequency of up to 107 Hz and measured the graphene’s resistance to show that the device’s ferroelectricity performance did not degrade. They found that the devices remained robust after more than 1011 switching cycles.
Immobile charged defects
Meanwhile, a team led by Fucai Liu of the University of Electronic Science and Technology of China, in collaboration with colleagues at Ningbo Institute of Materials Technology and Engineering (NIMTE) of the Chinese Academy of Sciences, Fudan University and Xi Chang University, demonstrated a second fatigue-free ferroelectric system. Their device was based on sliding ferroelectricity in bilayer 3R-MoS2 and was made by sandwiching this material between two BN layers using a process known as chemical vapour transport. When the researchers applied pulsed voltages of durations between 1 ms and 100 ms to the device, they measured a switching speed of 53 ns. They also found that it retains its ferroelectric properties even after 106 switching cycles of different pulse durations.
Based on theoretical calculations, Liu and colleagues showed that the material’s fatigue-free properties stem from immobile charged defects known as sulphur vacancies. In conventional ferroelectrics, these defects can migrate along the direction of the applied electrical field.

‘Slidetronics’ makes its debut
Reporting their work in Science, they argue that “it is reasonable to assume that fatigue-free is an intrinsic property of sliding ferroelectricity” and that the effect is an “innovative” solution to the problem of performance degradation in conventional ferroelectrics.
For their part, Yasuda and colleagues, whose work also appears in Science, are now exploring ways of synthesizing their material on a larger, wafer scale for practical applications. “Although we have shown that our device is promising for applications, we have only demonstrated the performance of a single device until now,” Yasuda tells Physics World. “In our current method, it takes many days of work to make just a single device. It is thus of critical importance to develop a scalable synthesis method.”