Good science communication is not just about explaining your work. As Brian Clegg argues, it’s about being able to put yourself in a non-scientist’s shoes to anticipate the kind of questions they will want to have answered
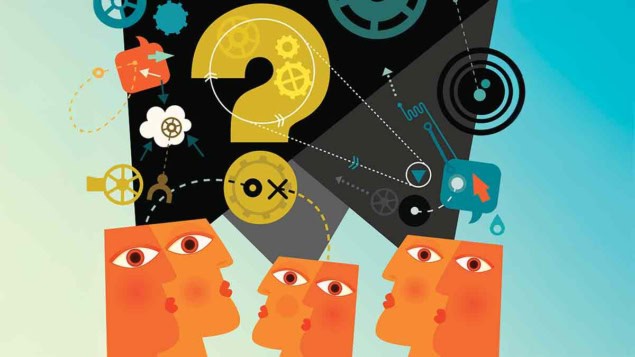
From books and lectures to blogs and social media, today’s physicists are expected to do far more public communication than their predecessors. However, communicating science well is far from easy. Obstacles include the comfortable familiarity of jargon and a natural distaste for the imprecision required by simplification. But there is a more fundamental barrier, which arises from scientists and non-scientists speaking different languages and having different mental models of how the world works.
In my career as a science communicator, I have observed countless online exchanges between scientists and the public, I’ve read and reviewed more than 1000 popular-science books, written 26 of my own, and spoken to numerous readers and attendees at public lectures. My conclusion is that physicists find communication hard because their idea of the questions physics is trying to answer is so different from those of their readers and the wider public. Although physicists aren’t unique in these problems, the concepts they need to communicate are often less immediately accessible than those from biology, chemistry and other fields.
“Why” versus “how”

Can we unify quantum mechanics and gravity?
The scientist–public language barrier was perfectly highlighted in a blog post written last September by Sabine Hossenfelder, a theoretical physicist and research fellow at the Frankfurt Institute of Advanced Studies (ow.ly/NsJO3077wZc). Hossenfelder puts a lot of effort into communication, not only through her Backreaction blog but also interacting via social media and writing for magazines, including Physics World (see “Can we unify quantum mechanics and gravity?” October 2013 pp42–43). She is anything but an ivory-tower physicist.
In her post, Hossenfelder analyses a question-and-answer discussion on Discover magazine’s website last September between staff editor Bill Andrews and a reader called Jeff Lepler from Michigan in the US. “Are we,” wonders Lepler, “any closer to understanding the root cause of gravity between objects with mass?” To which Andrews replies: “Sorry, Jeff, but scientists still don’t really know why gravity works. In a way, they’ve barely just figured out how it works.”
To the public, science is about the search for simple truths that explain how nature functions and, as a result, possibly also getting some idea of the why
In response, Hossenfelder asks “What’s that even mean – scientists don’t know ‘why’ gravity works?” She asks this rhetorically, going on explain that why gravity works is not a scientifically meaningful question; it’s rather how gravity works that physicists aim to answer.
It has only been since the development of modern science, however, that scientists have concentrated on answering how something works. In the time of natural philosophy – the precursor to modern science – scholars did indeed attempt to answer the question why. For this reason, some modern scientists write negatively in their popular books about the physics of, say, Aristotle and his contemporaries. But the natural instinct of Ancient Greek philosophers – shared by many people today – was to be driven by the search for causal reasons. Aristotle’s explanation of gravity depended, in other words, not on how things were attracted to the centre of the universe, but rather why this happened.
Aristotle believed that the four elements (earth, air, fire and water) each had their own natural tendencies. The reason why, for instance, an Earth-based object like a rock fell, he argued, was because its natural place was the centre of the universe (which meant the Earth). Aristotle tried to explain why the rock fell, not how it fell. Modern scientists can easily dismiss the philosopher’s explanation as irrelevant. But if they don’t understand the thought process behind the question, they miss a natural line of thinking for a non-scientist, which needs to be addressed rather than dismissed.
More fundamental for Hossenfelder than the language niggle, though, was the suggestion that scientists have “barely figured out how [gravity] works”. In her blog post, Hossenfelder responds that we do know how gravity works. “The purpose of science is to explain observations,” she writes. “We have a theory by the name General Relativity that explains literally all data of gravitational effects. Indeed, that General Relativity is so dramatically successful is a great frustration for all those people who would like to revolutionize science à la Einstein. So in which sense, please, do scientists barely know how it works?”
But answering the question “How?” itself merely generates a second-level question. If you said gravity works by warping space–time, the immediate response of a non-scientist would be: “Yes, but how does matter warp space–time?” And now physicists are stumped. They can’t answer that question, because repeatedly asking “How?” leads to a dead end.
Physicists may describe constructing a mathematical model as understanding, but it isn’t what most people mean by the word
To the public, science is about the search for simple truths that explain how nature functions and, as a result, possibly also getting some idea of the why. But when a physicist talks of understanding something, they have in mind being able to construct a model – usually mathematical – that matches, as closely as possible, the data that are observed. Physicists may describe this as “understanding”, but it isn’t what most people mean by the word. I feel it’s this lack of a shared vision between the public and the physics community that creates an inevitable barrier.
Offering lettuces to an ass
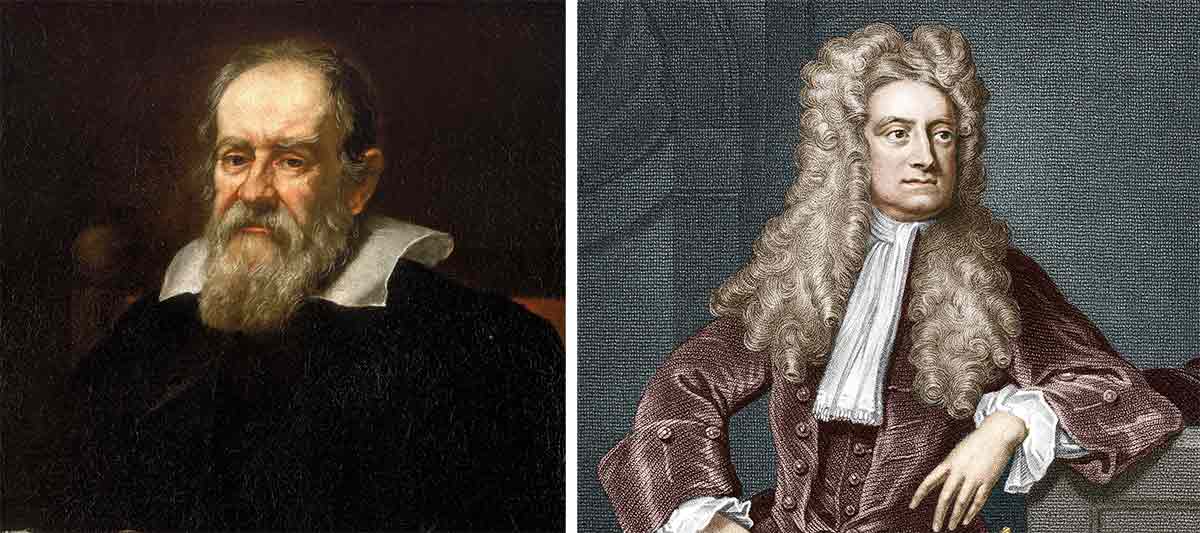
It can sometimes feel to the public that scientists are guilty of perpetuating the concept that wisdom should be kept from the masses. Writing in the 13th century, friar and proto-scientist Roger Bacon noted that Aristotle said “It is stupid to offer lettuces to an ass since he is content with thistles.” Bacon agrees, telling us that “The cause of obscurity in the writings of all wise men has been that the crowd derides and neglects the secrets of wisdom and knows nothing of the use of these exceedingly important matters.”
This philosophy comes through in Isaac Newton’s Principia, particularly when set alongside Galileo’s Two New Sciences. Each of these books is a masterpiece of physics. Yet the approach taken could not be more different. Galileo writes in his native language, Italian. His prose is accessible and his discussions of physical principles take the form of a three-way discussion between two experts (Salviati and Sagredo) and a third person, Simplicio. Presenting the voice of the ordinary man, Simplicio is the most interesting participant in this context. He asks the questions ordinary readers want answered, a simple device that enables Galileo to keep his text grounded.
By contrast, Newton writes in Latin, making his book less accessible to the non-expert. Indeed, Newton goes out of his way to make the book difficult to read. He notes that he originally intended the third part of the book, The System of the World, to be for a popular audience “so that it might be more widely read”. However, he then deliberately recast it to make it more obscure.
“Those who have not sufficiently grasped the principles set down here,” he remarks, “will certainly not perceive the force of the conclusions, nor will they lay aside the preconceptions to which they have become accustomed over many years; and therefore, to avoid lengthy disputations, I have translated the substance of the earlier version into propositions in a mathematical style, so that they may be read only by those who have first mastered the principles.”
Perhaps physicists should be a little more like Galileo and a little less like Newton when communicating with the public.
Delivering the goods
Getting a better feel for what the public’s questions mean isn’t the only prerequisite for good communication. Another danger is that if we take too much for granted, our explanations don’t deliver. In a recent book, A Farewell to Ice (2016 Allen Lane), Peter Wadhams – a sea-ice scientist and former professor of ocean physics at the University of Cambridge – tries to explain the concept of Fourier analysis to the reader. “The Fourier series,” he writes, “by which any function can be split into a set of harmonics…” – without thinking that anyone who knows what a function being split into harmonics involves probably knows about Fourier analysis. It’s a non-definition – a trap that many communicators fall into.
However, there is a further barrier when it comes to understanding and anticipating the questions the public might ask. When scientists are writing for the public they need to bear in mind the kind of questions that their words will generate in the mind of the reader and be prepared to answer those questions. Paradoxically, whereas using too many technical terms over-complicates an explanation, a lack of anticipation leads to over-simplification.
As an example, in his otherwise excellent book Neutrino Hunters (2013 Scientific American), Ray Jayawardhana – an astrophysicist at York University in Canada – describes the challenges facing people who build detectors for these elusive particles. Jayawardhana introduces us to a development of the Japanese Super-Kamiokande detector: “Beacom and his colleagues have suggested that dissolving a bit of gadolinium, a silvery-white metal, in the giant water tank at Super-Kamiokande would do the trick [of distinguishing supernova relic neutrinos], since the fix would enhance the detector’s sensitivity to relic neutrinos.” Then he moves on. Gadolinium is not mentioned again.
In that extract, we see Jayawardhana answering a potential reader question – “What is gadolinium?” – though, to be frank, giving the element’s colour feels a bit like asking what kind of car someone has and being told that it’s blue. However, the author leaves the reader mentally stranded. Jayawardhana knows the answers, but doesn’t share them. As a result, the reader is mired in questions that are never answered. How does gadolinium improve detection? How does this enable the detector to distinguish relic neutrinos from common-or-garden local ones? We don’t find out.
What is lacking here is an ability to pre-empt questions that the reader may have in response to a piece of writing, and then to formulate a response with which the lay person will be satisfied. Let me use one more example to show this barrier in action.
Mind the warp
A few years ago, I was researching my popular-science book Gravity (2012 St Martin’s Press). In the section on the general theory of relativity, I had to get across how it is that the warping of space–time produced by matter can lead to the effects that we experience as the force of gravity. Inevitably I wheeled out the “bowling ball on a sheet of rubber” analogy, which uses a flat sheet of rubber, stretched taut, as a 2D model of space.
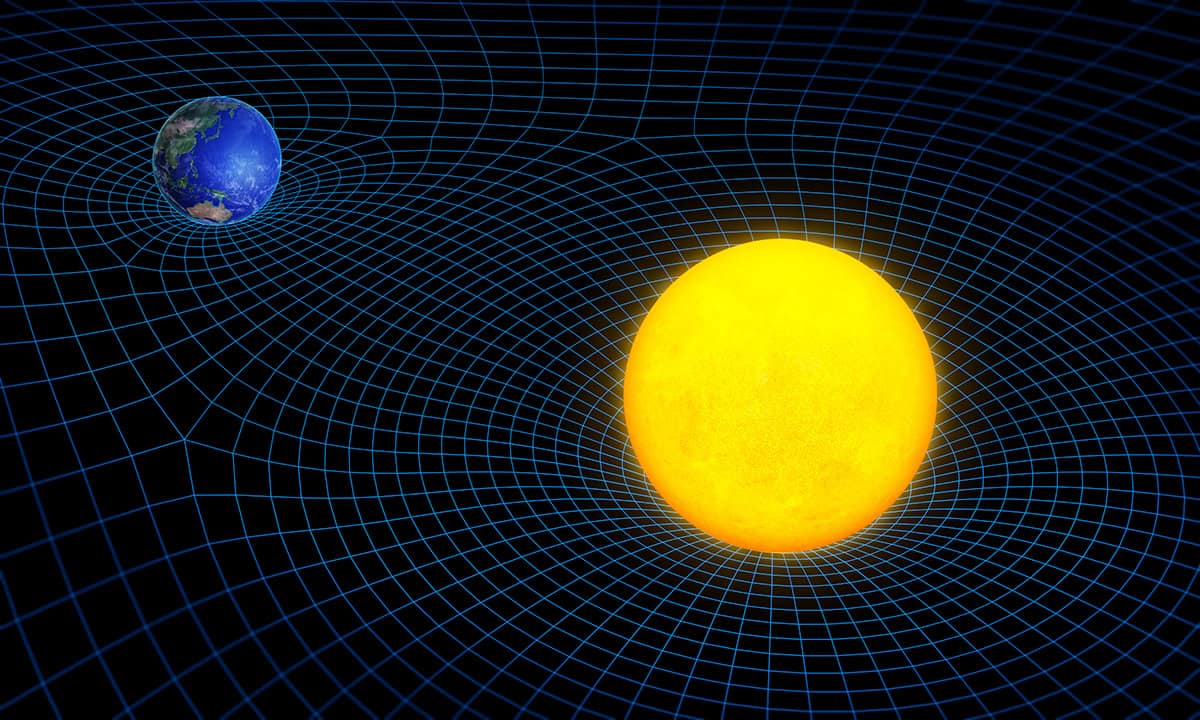
We imagine a beam of light, or the straight-line motion of a planet flying free through space, as a coloured line on the surface of the sheet. We then place a bowling ball on the sheet and it distorts the rubber. As a result, the line is no longer straight. It curves around the ball. This, we say, is rather like the way that matter distorts space (though in 3D, rather than 2D), causing light and the straight-line motion of celestial bodies to bend around massive objects like stars and planets. At this point, traditionally, the popular account of gravity moves on. But there is a question that is not asked. To take Newton’s example: how does this make an apple fall?
If this is ever mentioned when using the rubber-sheet analogy, we usually get some hand-waving suggestion that the apple slides down the indentation in the rubber sheet towards the massive object. But what makes the apple move? Gravity – so we haven’t got anywhere. Because I couldn’t come up with a sensible extension to the rubber-sheet analogy, I e-mailed a wide range of physicists asking how they would explain, for the general public, how the apple goes from being stationary to falling.
Most academics didn’t respond. This might seem unsurprising, but, on the whole, professional scientists are happy to answer meaningful queries. The apparent implication here is that either the rubber-sheet analogy has been stretched too far or that the answer is just too complicated for little minds to worry themselves with. Where I did get replies, they tended to suggest my question was irrelevant or, well, the mathematics works, so let’s not worry about the analogy. A typical argument from those in the “irrelevant” camp” was: “A force is a force, of course, and asking why/how it causes an acceleration already seems a bit circular.” Asking how you can have a force producing action at a distance is not at all circular, but something that has worried people for a long time. There still needs to be a cause.
Finally, I got an explanation I could use from Friedrich Wilhelm Hehl of the University of Cologne in Germany. His initial explanation, admittedly, had limited value for the public: “As you correctly say, in the ‘bowling ball on a rubber sheet’ picture, the sheet represents space–time. In space–time the 4-velocity of a particle is just the tangent unit-4-vector of the path traced by the particle. Since a curve in the sheet at a certain point has always a tangent 4-vector, the particle has to follow the path with the corresponding 3-velocity.”
This was useful to know, I’m sure, but didn’t exactly illuminate things for the non-physicist. But after a little probing, Hehl translated this explanation into a more amenable form. By getting the reader to think not of distorting space but space–time, and thinking through the implications of warping the time dimension, which with nowhere else to go has to produce a change in the space dimensions, it was possible to extend the analogy to see how warped space–time could produce motion.
Lessons to learn
Altogether, I’d suggest that there are three significant lessons from these examples. First, if you get a question that doesn’t make sense to you, don’t simply assert that it is a silly question. More likely, it’s the scientist who is at fault for not understanding – not the questioner for being dumb. Ask for more detail. And if you still don’t get it, ask a friend who isn’t a physicist what it means. (If you don’t have any friends who aren’t physicists, get out more.) Ensure your answer takes into account the questioner’s viewpoint.
Try to break down what you are saying and identify where the assumptions are
Second, when you are explaining something, always try to be aware of your assumptions about what the questioner already knows. This can be difficult, because so much of what you assume has been part of your working life for so long. Try to break down what you are saying and identify where the assumptions are – then make sure that they are ones that hold for your audience too. If not, deal with them.
And finally, don’t over-simplify. We are used in academic writing to ensuring that statements are backed up by sources or experimental evidence. In public communication, it’s important to back up statements with explanations that the reader needs (and not just the explanations that you think you need). Provided you do explain appropriately, you can go into more detail than you might think. Many books on gravity for a lay audience don’t include Einstein’s field equations. Mine did – not because I felt the reader is ever going to do the maths, but to satisfy their curiosity of what these things look like and to explain the main elements of the equations, without straying into mathematical complexity.
If physicists are to communicate well with the public, we need to do more than just weed out jargon and simplify complex mathematics. We also need to understand the nature of the public’s questions, anticipating queries that are based on a different kind of thinking. It’s a stretch, but it is possible – and, I would argue, is essential if we want the public, which controls the purse strings, to support progress and advancement in physics.