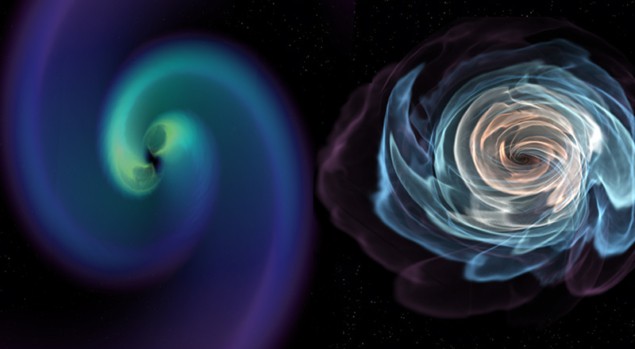
Astronomers have made one of the biggest breakthroughs of the decade after detecting both gravitational waves and gamma rays from the merger of two neutron stars. Announced today at a co-ordinated set of media briefings in Washington DC, London, and elsewhere, the detection was made on 17 August, with the gravitational waves spotted by the LIGO–Virgo collaboration and the gamma rays picked up by the Fermi Gamma-ray Space Telescope. The observations prompted astronomers to point dozens of different telescopes and detectors around the world, and in space, at the origin of the signals in a distant galaxy. Together, these facilities captured radiation from the aftermath of the merger across the electromagnetic spectrum from gamma rays to radio waves.
As well as being the first-ever example of “multimessenger astronomy” involving gravitational waves, the observations have yielded important clues about how heavy elements, such as gold, are produced in the universe. The ability to measure both gravitational waves and visible light from neutron-star mergers has also given a new and independent way of measuring the expansion rate of the universe. In addition, the observation settles a long-standing debate about the origin of short, high-energy gamma-ray bursts.
Given the far-reaching impact of the new measurements, rumours were circulating for weeks in advance of today’s announcement. Sheila Rowan, director of the Institute for Gravitational Research of the University of Glasgow in the UK and a LIGO team member, is thrilled at “the sheer versatility” of the observations, saying that “exciting science has already been done with many firsts being reported”.
Up all night
The gravitational-wave observation, dubbed GW170817, is the loudest ever seen in both US-based LIGO gravitational-wave detectors, which are in Hanford, Washington, and Livingston, Louisiana. A somewhat weaker signal was seen by the Virgo gravitational-wave detector near Pisa, Italy. Virgo had on 14 August detected its first gravitational wave from a black-hole merger, which not only told scientists that the detector was working properly but also suggested that the GW170817 signal came from a direction that Virgo is least sensitive to.
The merged neutron stars seen in the GW170817 event likely formed a black hole with a powerful jet, which produced the gamma rays that were also observed. Information from the three detectors enabled the LIGO–Virgo team to limit the location of the merged neutron stars to about 28 square degrees of sky. A battery of telescopes and other instruments was then able to pinpoint the source to galaxy NGC 4993, which lies about 130 million light-years from Earth.

Among those involved in the search was Nial Tanvir from the University of Leicester in the UK, who says that astronomers immediately triggered observations on several telescopes in Chile to search for the explosion that they expected the merger to produce. “In the end we stayed up all night analysing the images as they came in, and it was remarkable how well the observations matched the theoretical predictions that had been made,” he says.
The gravitational-wave signals were measured for about 100 s, whereas those from previous black-hole mergers lasted less than about 1.5 s. This longer measurement time reduced the uncertainty in the location of the merger, while the shape of the signal allowed astronomers to estimate the masses of the neutron stars to each be about 1.1 to 1.6 solar masses. The amplitude of the signal gives the distance to the source to within a 30% margin of error.
High-energy gamma rays from GW170817 were detected in the form of a short burst, some 2 s after the gravitational waves. Astronomers had suspected that such bursts are caused by neutron-star mergers, but had little understanding of how it happens. “We confirmed that colliding neutron stars power short gamma-ray bursts, solving one of the greatest mysteries in present-day high-energy astrophysics,” says Francesco Pannarale of the University of Cardiff in the UK.
The prompt arrival of the gamma-ray signal also confirms that gravitational waves travel at the speed of light, while the ability to observe light and gravitational waves arriving from distant objects will allow physicists to perform more stringent tests of Einstein’s general theory of relativity.
Neutron matters
The gravitational waves produced in GW170817 were given off by the two neutron stars as they spiralled inwards. As stars approached each other, their shapes can be distorted by tidal forces. The amount of distortion depends on the state of matter in the star and how the matter is distributed. Such distortions would affect the gravitational-wave signal, but were not seen in GW170817. Mark Hannam, who is also at Cardiff, says that this has allowed astrophysicists to already rule out certain models of what that matter could be like.
Unfortunately, the LIGO–Virgo collaboration was unable to detect gravitational waves from the precise moment the neutron stars merged, as the frequency of those waves was too high to be seen by the detectors, meaning that the gravitational-wave astronomers were unsure if the pair formed a neutron star or a black hole. According to Jim Hough of the University of Glasgow, there are four likely scenarios. One involves the two neutron stars merging to form another neutron star. Another is that a “hypermassive neutron star” could be formed, which would decay in less than a second into a black hole. The third option is the creation of a “supermassive neutron star” that decays into a black hole within a few hours. The final possibility is the direct formation of a black hole.

Subsequent observations by as many as 70 other telescopes, however, suggest that the ultimate result of the merger was a black hole surrounded by an accretion disc of material. As this material was sucked into the black hole, a fast-moving jet of material blasted outward along the black hole’s axis of rotation. When this jet collided with gas in the galaxy, it started slowing down and the lost kinetic energy was broadcast as gamma rays. Because Earth is roughly in the same direction as the GW170817 jet, astronomers were able to detect those rays.
As it moved outward from the black hole, the jet slowed down and the energy of the emitted radiation dropped too. This explains why emissions of lower-energy X-rays, visible light, infrared and radio waves were also all detected in the weeks after 17 August. Indeed, astronomers are still observing signals from GW170817 two months on.
Forging heavy elements
One important early result of seeing GW170817 – according to Imre Bartos of the University of Florida in the US – is evidence for the creation of elements heavier than iron in the neutron-star merger. Such elements are believed to be created within neutron-rich heavy nuclei ejected during the merger. These nuclei then radioactively decay to stable heavy nuclei, and the radiation given off causes the surrounding ejecta to glow. This glow was first detected in GW170817 by the relatively small Swope Telescope in Chile. “Then after some initial observations the big guns came in and took very detailed measurements that gave us a lot of information,” says Bartos. “These include the US Gemini Observatory, the European Very Large Telescope and NASA’s Hubble Space Telescope.”
Kate Maguire of Queen’s University Belfast in Northern Ireland says that astronomers have discovered that this neutron-star merger scattered heavy chemical elements, such as gold and platinum, out into space at high speeds. “These new results have significantly contributed to solving the long-debated mystery of the origin of elements heavier than iron in the periodic table,” she says.
Look back in time
These spectacular observations have also given astronomers a new way of measuring the Hubble constant, which is the rate of expansion of the universe as a function of distance from Earth. Indeed, Hough points out that obtaining such a technique was one of the initial reasons why LIGO and its European precursor GEO were built. The measurement is made possible because knowing the amplitude and polarization of a gravitational wave lets astronomers determine the distance to its source. And being able to see the galaxy of origin, they can determine the redshift of its light, and hence the velocity it is moving away from Earth. Based on these distance and velocity measurements, the resulting Hubble constant calculated from GW170817 agrees with independent observations.
“As gravitational-wave detectors improve,” says Hough, “we will be able to look further into the past to measure the Hubble constant.”
After just two months of observation, GW 170817 is already one of the most studied object outside of the solar system, Bartos told Physics World. “We are still learning a lot and data will be analysed for years to come.” Perhaps more importantly, Bartos does not see this as a singular or rare observation. “We will,” he says, “see many more of these events in the future.”
The observations are described in a number of papers published in The Astrophysical Journal Letters, Physical Review Letters, Nature and Science.
- There is much more about neutron-star mergers and other cosmic events that can be studied by LIGO–Virgo in the Physics World Discovery ebook Multimessenger Astronomy