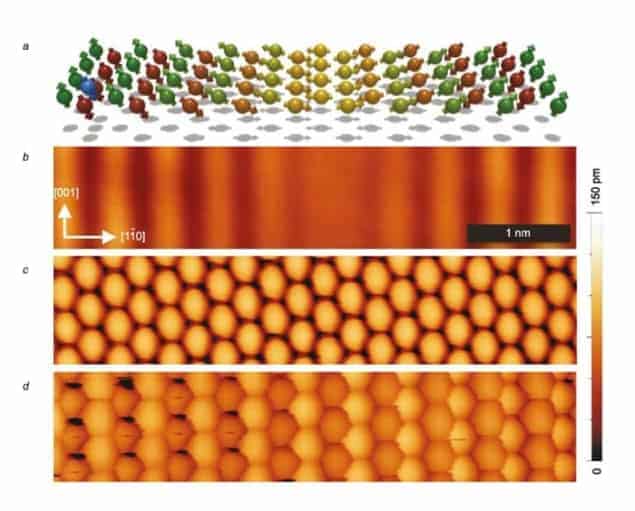
An international team of researchers says that it has measured the spin-dependent component of friction of a single atom as it slides across a magnetic surface, for the first time. The team used a combination of spin-polarized scanning tunnelling microscopy and single-atom manipulation to push individual magnetic atoms over a magnetic template. Comparing experimental results with simulations, the researchers were able to conclusively say that spin-dependent friction contributes substantially to the overall frictional force experienced by an atom. They say that their results provide an essential step towards developing a fundamental theory of friction.
Although friction is one of the most pervasive forces in the universe, and is one of the oldest-known physical phenomena, the intricacies of the fundamentals of friction and its origins have eluded scientists. The study of friction, or “tribology”, looks at surfaces in contact from the macro to the nanoscale. A basic issue is to single out and connect an observed frictional force between two contacting materials with the physical, chemical and mechanical properties of the materials that cause the friction. But this is easier said than done.
Spinning free
Over the years, scientists have determined two factors that contribute substantially to friction at the macro level – namely, electronic degrees of freedom and lattice vibrations. “So if the electron system in the atom plays such a crucial role in friction, it is clear that we must consider spin too, as an electron possess not only charge but also spin,” explains Roland Wiesendanger of the University of Hamburg, Germany, who is one of the authors of the new work. He, along with lead author Boris Wolter and colleagues, has been interested in spin-dependent phenomena at the atomic scale for more than 25 years and has found very little experimental interest in spin friction. “This is probably because it is very difficult to find a system that allows us to identify spin friction easily,” says Wiesendanger. If one considers a ferromagnet, such as iron, for example, all the magnetic moments or spins are perfectly parallel, “so it is virtually impossible to distinguish between spin-dependent and normal frictional contribution”, Wiesendanger explains. “You must design a model system where the electronic properties are different from the magnetic properties,” he says.
That is precisely what the team did – it considered using an anti-ferromagnetic material, wherein neighbouring spins always point in opposite directions. In fact, the researchers created a manganese-on-tungsten-substrate material that is anti-ferromagnetic on the small scale and shows a spin-spiral state of about 170° between adjacent rows on the larger scale. (See image part (a)). They then studied the friction of this system by using a spin-polarized scanning tunnelling microscope (SP-STM) with a cobalt (Co) atom at the tip that slides over the manganese layer, with the Co atom moving from one lattice site to the next. The spins in the anti-ferromagnetic manganese layer are alternating, and the Co atom’s spin aligns with the nearest manganese atom, giving an alternating pattern. Bright and dark lines indicate atomic rows with parallel and antiparallel magnetization components relative to the tip magnetization.
Polarized tip
An SP-STM tip is used because if a normal metal tip was used to drag the Co across the manganese surface, the image shows only the symmetry of the atom lattice (see image part (c)). But when the team used a spin-polarized tip, it showed the weak magnetic coupling to the cobalt, and it is this image that reveals the magnetic order of the structure, showing the spin-dependent component. “Another appealing point about the magnetic manipulation imaging technique is the extremely high spatial resolution and large magnetic corrugation, although at the cost of slightly increased experimental and analysing effort,” says Wolter. “The first experimental discovery was a little bit of serendipity while working on SP-STM manipulation experiments with the sample, and the technique was then refined to improve the quality of the images for further study,” he explains.
To confirm the fact that the spin degree of freedom contributes towards the friction of the system, the team then carried out Monte Carlo simulations, using a system very similar to the experimental one. The simulated systems agreed. “It was the choice of the right model system that gave us the right to say that the spin-dependent component is definite,” says Wiesendanger. He further explains that the magnitude of the spin-friction contribution can be of the same order as that of the electronic or lattice vibration-dependent friction, and this is surprising considering the orders of magnitude of difference between chemical and magnetic coupling energies. They also expect the spin friction to be a general phenomenon occurring in a large class of systems, not just magnetic systems.
Model behaviour
The researchers’ findings are relevant for many reasons. First, that they have helped to better understand the fundamentals of friction, and their study serves as a starting point towards gauging the importance of the spin degree of freedom in “surface phenomena” such as the diffusion of magnetic atoms on magnetic substrates. On a more practical level, their work is relevant for magnetic data-storage systems such as hard drives, or magnetic motors with sliding parts.
“What is also very important is that our work,” says Wiesendanger, “demonstrates how important it is to find and build the proper model systems that give clear answers. The idea [of magnetic spin friction] has been around theoretically for a few years, but to pin it down, the model was essential, and this is equally important in all fields of physics.”
The research was published in Physical Review Letters.