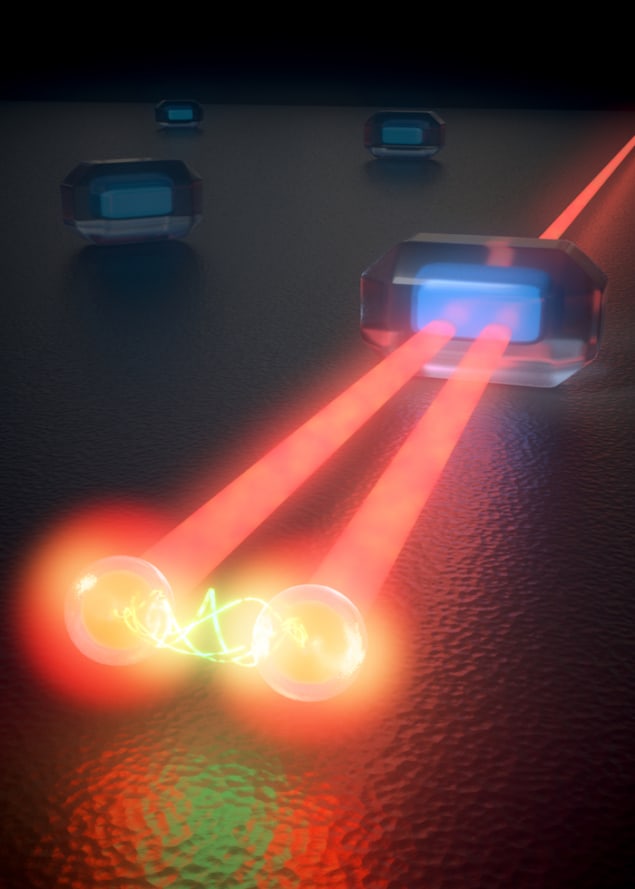
A new technique for imaging single molecules that does not rely on fluorescent emitters could find a host of applications in nanotechnology, photonics and photovoltaics. The technique, which was developed by researchers in Barcelona, works by detecting stimulated emission from single quantum dots at room temperature. Its speed makes it possible to trace charge-carrier populations through the entire absorption and emission cycle.
Single-molecule imaging techniques are widely employed in biology. To date, they have been entirely based on detecting spontaneous fluorescence from the sample being imaged. In these fluorescence-based techniques, researchers typically excite the sample at wavelengths at which it absorbs light and then detect redshifted (lower energy) fluorescence signals. This makes it relatively simple to block the background light from the excitation beam and detect only the fluorescence.
However, fluorescence imaging is far from perfect, since it is limited to molecules that fluoresce efficiently. Fluorescent light is also both incoherent and prone to “bleaching”, where the signal fades after the molecule can no longer fluoresce. A third drawback is that spontaneous emission is a relatively slow process, occurring on a timescale of nanoseconds. This means that fluorescence-based imaging can only provide information on the lowest excited state of the target molecule, since more highly excited states have shorter lifetimes on the order of femtoseconds or picoseconds.
Detecting stimulated emission
Techniques based on detecting stimulated emission (SE) offer several advantages. All molecules exhibit SE, even those that do not fluoresce. SE also avoids bleaching, since the molecule spends little time in the excited state, and is much faster, as the light is emitted on femtosecond timescales – meaning that SE can provide information on the dynamics of excited states. The downside is that the laser beam that drives the stimulated emission also produces a significant amount of background light.
Researchers at the ICFO in Barcelona, along with Lukasz Piatkowski and colleagues in Niek van Hulst’s group at ICREA, have now overcome this issue by using ultrashort laser pulses to image individual colloidal nanocrystals, or quantum dots (QDs). By applying the laser pulses, the team showed they could force individual QDs (single colloidal CdSe/CdS rod-in-rods made in Iwan Moreels’ group in Ghent) to emit through a SE process, rather than waiting for them to spontaneously emit light.
The researchers begin by using a laser to “pump” the QD into a highly excited state in the conduction band. Afterwards, the excited charge carriers (electrons and holes) decayed through the excited state manifold, eventually ending up in the lowest exited state (the band edge) in the core of the nanostructure. The scientists then apply a second (probe) laser pulse at the resonant frequency of the core band-edge transition. This makes the charge carriers recombine, causing the QD to relax back to the ground state and producing a photon via stimulated emission. Because this emitted photon is in phase with the one that stimulated it, all the light produced is coherent.
Technique beats the limit of the background signals
Piatkowski explains that the team’s technique works because the probe pulse train used to modulate the excited state population operates at high, MHz, frequencies, allowing for phase sensitive detection (using a lock-in amplifier) and beating the limit of the background signals. The pump and probe pulses are also synchronized because they derive from the same broadband laser, which means that the researchers detect stimulated photons on top of a very intense stimulation beam. The stimulation beam is therefore a background signal to the stimulated emission signal. The technique is ultrafast, too and works on the femto- to picosecond scales, which allows the researchers to image their QDs at any moment during the photocycle.

A century ago Einstein sparked the notion of the laser
Thanks to this work, which is detailed in Science, the researchers have started to investigate electron-hole core-shell dynamics in their QDs. Such studies will make it easier to understand and engineer trap-free, non-blinking, photorobust QDs for future applications in optoelectronics.
Extensions to biology
Studies of biological systems could also benefit, van Hulst says, since the ultrafast response will allow researchers to study the dynamics of important energy transfer systems in biological cells. “At ICFO, we are investigating coherent energy transfer in photosynthetic light-harvesting complexes (which are used by plants to enhance collection of incoming light),” Piatkowski says. “The new technique could allow us to do this on the single light-harvesting complex level.”
The ICFO team says that it would now like to extend its technique to molecules and biomolecular complexes. “We are also working on three- and four-pulse schemes to combine 2D-spectroscopy with SE and luminescence detection,” van Hulst tells Physics World. “Each technique will complement the other and provide different information: luminescence will report on the ground state of a molecule while SE its ultrafast excited state dynamics.”