According to the physicist Charlie Ironside, our ability to deal with future deadly pandemics could be better – if we look to the far-ultraviolet. Jon Cartwright reports on a call to arms for the LED industry
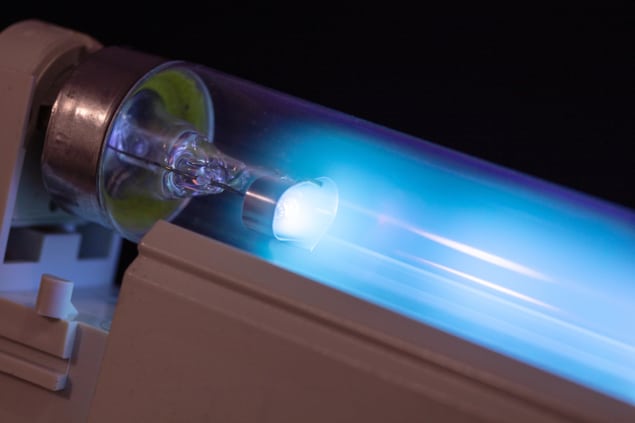
Imagine a world where people travel as they wish. They shake hands when they make new acquaintances, embrace when they greet close friends and elderly relatives. They do not bother to laboriously disinfect their work surfaces, or wash their hands once they have dealt with the post. They go shopping as they please and find no shortage of provisions. They work in offices, laboratories, shops, restaurants and building sites. They conduct meetings in person, and think nothing of it when they jet off to their favourite holiday destination. They do all this because a COVID-19 vaccine has been developed, rolled out and administered to the entire populace, making all the chaos of 2020 a distant memory. Everything is back to normal.
This is the ending to the coronavirus pandemic we are all hoping for, and, give or take some of the details, there is no reason why it is not possible. But even in this optimistic scenario, there is a deep fear among scientists and policy makers: what happens next time? For if there is one lesson that COVID-19 has taught us, it is that our modern lifestyles are fatally ill-suited to the emergence of novel viruses – and novel viruses there will always be. Any drugs and vaccines we develop for COVID-19 will be ineffectual against the next viral pandemic, which may well consist of a different family of virus altogether. Indeed, unless anything in our approach to pandemics changes, the next one will entail another psychologically and economically crippling lockdown while scientists find a cure – however long that takes.
Yet according to one scientist, there is something we can do differently next time. Charlie Ironside of Curtin University in Perth, Australia, is not a virologist or an epidemiologist but a physicist – one who has spent 30 years specializing in semiconductor optoelectronics. His solution: far-ultraviolet light-emitting diodes (far-UV LEDs).
A narrow range of far-UV wavelengths seems to be safe for humans, while being lethal for viruses. Sterilization could become easy, routine and effective
To avoid any misunderstanding, UV light is, on the whole, incredibly dangerous, and people should never seek exposure to it. However, there is emerging evidence that a narrow range of far-UV wavelengths is safe for humans, while being lethal for viruses. If LEDs could be mass-manufactured with this sweet-spot in UV emission, explains Ironside, they could be integrated into everyday lighting and consumer technology for pandemic control. Sterilization could become easy, routine and effective, he says, preventing new infections while allowing many aspects of ordinary life to continue. “It could flatten the curve of new infections without so much social distancing,” he adds. Lockdowns may not be necessary.
Ironside is referring to his proposal as a “call to arms” for LED researchers and the semiconductor industry as a whole. But is it realistic?
Antique weaponry
For more than a century, UV light – which consists of photons of wavelength 200–400 nm – has been known to kill bacteria and viruses. As a result, it is already in our arsenal against COVID-19 – or more accurately SARS-CoV-2, the novel coronavirus from which the current severe respiratory disease emerges. UV floodlights are installed in hospitals to sterilize the air and horizontal surfaces, or inside trays to sterilize medical instruments. Handheld UV torches sterilize where floodlights cannot reach. In China, buses are even parked at night in UV-illuminated depots. Where UV facilities are not already installed, robotic trolleys carrying UV lamps are sent into rooms via remote control.
While effective, there are two major disadvantages with these technologies. The first is that the UV light is usually emitted by fluorescent tubes, which are big, fragile and unwieldy for all but specialist applications. The second, bigger disadvantage is the effect of UV radiation on humans.
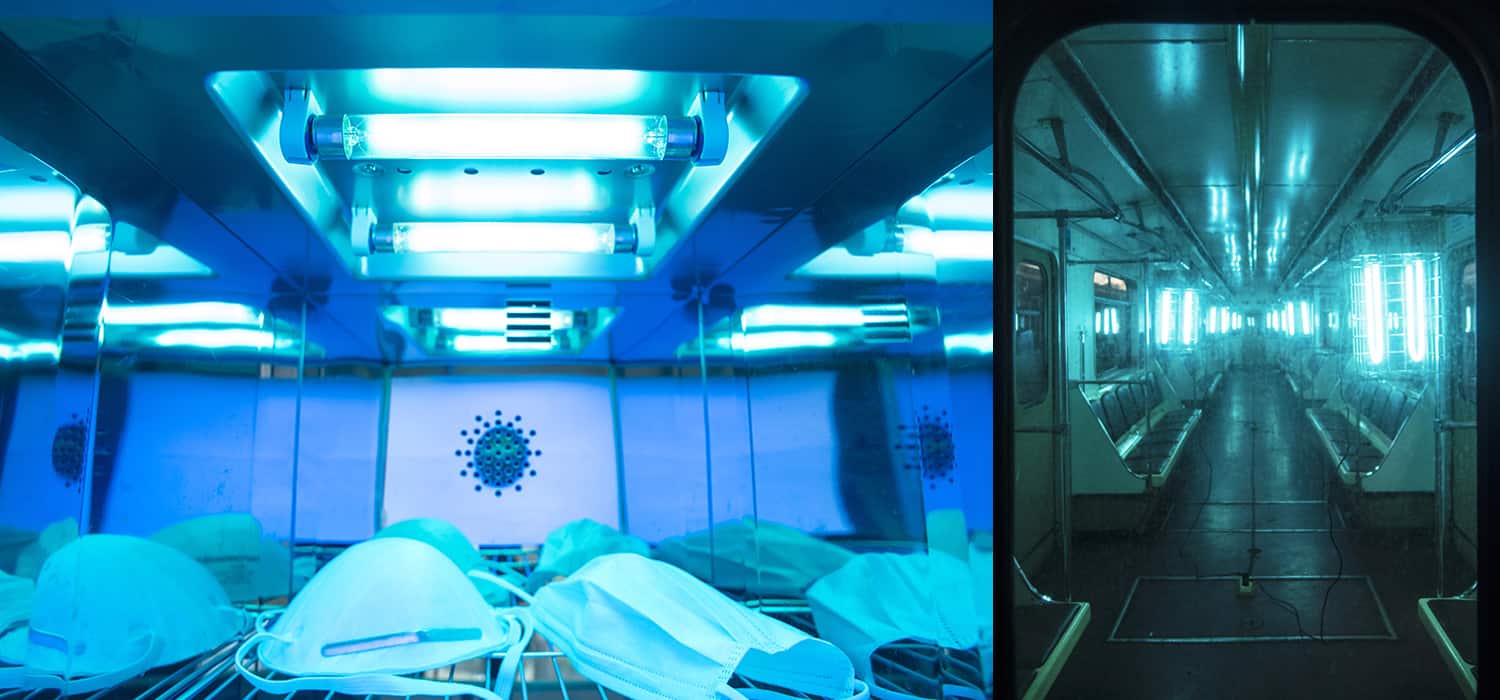
We are all familiar with the first two bands of UV – UVA (315–400 nm) and UVB (280–315 nm) – as these are components of sunlight that filter through our atmosphere. Both cause sunburn – UVB more so – and, in the worst cases, skin cancer. But we rarely encounter UVC (200–280 nm), because it is absorbed by the Earth’s ozone layer. Not only does UVC cause terrible sunburn, it is also supremely effective at destroying DNA, making human exposure to it highly dangerous. Unfortunately, the wavelength emitted by UV fluorescent tubes is usually around 250 nm – right in the middle of the UVC band. For that reason, UVC germicidal lamps cannot be operated with anyone in their firing line, a fact that greatly limits their application in times of pandemics; after all, the risk of infection is greatest when people are living or working in close quarters, such as hospitals. In April the International Ultraviolet Association and RadTech North America – two educational and advocacy organizations consisting of UV equipment vendors, scientists, engineers, consultants and health workers – issued a joint statement to remind the public that there is no accepted safe means of exposing the human body to UV to kill viruses (see analysis box below).
But not all wavelengths of UVC are as damaging as others, as a group of researchers led by physicist David Brenner at Columbia University in New York, US, showed in 2017. Their research relied on an excimer lamp – a type of light tube containing molecules, or excimers, that can briefly exist in an excited electronic state before returning to their ground state, and in doing so emit UV radiation at various wavelengths in the UVC band depending on the molecules used. On exposing mice to 222 nm, far-UVC light from a krypton-chlorine excimer lamp, Brenner and colleagues found no evidence of skin damage, even though they found that the same light was effective at killing the superbug MRSA (Radiat. Res. 187 493).
The result was corroborated a year later by Kouji Narita at the Hirosaki University Graduate School of Medicine in Japan and colleagues. This team also confirmed that the 254 nm-wavelength emission of a conventional germicidal lamp did induce sunburn-like skin damage (PLOS One 13 e0201259). That same year, Brenner and colleagues found that 222 nm light is able to destroy airborne viruses as well. In their test, with an exposure of just 2 mJ/cm2, the far-UVC radiation safely inactivated more than 95% of airborne H1N1 influenza, the virus behind the 2009 swine flu pandemic (Sci. Rep. 8 2752). There is even evidence that far-UVC light is safe for the eyes: last year, Sachiko Kaidzu of Shimane University in Izumo, Japan, found no damage to the corneas of rats exposed to 222 nm electromagnetic radiation (Free Radic. Res. 53 611).
The reason for the lack of skin damage from far-UVC light, according to Brenner, is simply down to the range of absorption in biological materials (see box above). Being a shorter wavelength than other UVC light, far-UVC photons are barely able to penetrate the skin’s outermost layer of dead cells, which is often tens of microns thick. On the other hand, it can still easily penetrate bacteria and viruses, which are usually less than 1 μm thick. As Brenner said in a TED talk in late 2017, “I’m thrilled that we’ve now got a completely new weapon against superbugs” – and, he later noted, viruses. (Brenner did not respond to requests from Physics World for an interview.)
Ultraviolet: the invisible killer
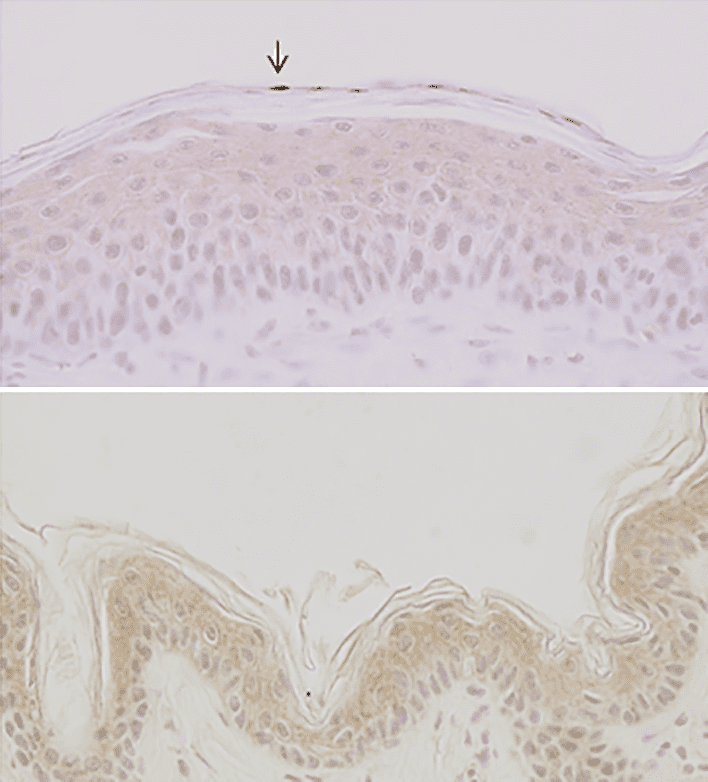
Physicians suspected as far back as the late 19th century that there is a link between skin cancer and Sun exposure. However, it was only in around 1940 that scientists first realized that cell mutations studied in the lab went hand in hand with levels of ultraviolet (UV) absorption by DNA, and therefore that it was specifically UV radiation to watch out for. Much later they would discover, via modern DNA sequencing techniques, that those very same mutations are present in actual skin tumours, cementing the UV–cancer link.
But how does UV damage DNA in the first place? DNA is made up of four nitrogen-containing bases, one of which is thymine. When a thymine molecule absorbs a UV photon, one of its electrons is promoted to an unfilled orbital, making the molecule very reactive. In this instance, it can bond to another thymine molecule, forming a dimer. A special protein is able to repair such damage – so long as it is not too extensive. If it is extensive, most of the time the cell containing the DNA simply dies and you get sunburn; but sometimes the damaged DNA causes the cell to become cancerous, and grow and divide uncontrollably. This is the basis of a tumour.
To be absorbed by the electrons in thymine, however, UV radiation has to actually reach the DNA, and some wavelengths of UV stand more chance than others. That is because the 5–20 µm thick outer layer of “dead” skin, known as the stratum corneum, contains only proteins – no DNA-containing nuclei. The absorption spectra of proteins are well known. Below a wavelength of 250 nm, their absorption of UV light rises rapidly: it takes some 3 µm of biological tissue to reduce the intensity of 250 nm UV radiation by half, but just 0.3 µm for the same attenuation of 200 nm far-UVC. According to David Brenner at Columbia University, and colleagues, far-UVC is “drastically” attenuated before ever reaching the nucleus of a living cell, potentially making it safe for human exposure.
Big impact
Brenner’s work has gained a lot of attention, with articles about it appearing in Time, Newsweek, the Wall Street Journal and CBS News, among other outlets. It is easy to see why far-UVC light could radically improve our ability to deal with viruses, including those for which we have no cure. If 222 nm excimer lamps could be installed in or alongside existing light fittings, they could operate more or less continuously in public spaces such as hospitals, schools, train and bus stations, and airports – as well as on trains, buses and aeroplanes themselves – without any risk of harming people. Viruses such as SARS-CoV-2 spread in the air: if that air is irradiated, the viruses find it much harder to reach new hosts. Even without social distancing, people could minimize infections.
But all that will be possible only if the safety of 222 nm UV is proven beyond doubt. Peter Setlow, a molecular biologist at UConn Health in Farmington, Connecticut, US, is among those who would like to see longer-term studies of the effect of far-UVC on the skin, as the studies conducted so far have relied either on single doses or exposures over just a few hours. “The question is, exactly how much 222 nm UV penetrates the dead cells in the skin to get to those that are alive and working?” he says. “It seems the answer is certainly ‘not much’, but ‘much’ is not an absolute term. To do risk assessments, you would probably need to perform experimental tests on animals over a longer time, and also establish, for example, whether hospital gowns provide any protection against this UV light.”
Ironside agrees that the safety of far-UVC for humans needs to be comprehensively proven before it can be routinely used. But if its safety can be shown (and Ironside assumes it will), there will still be the problem that excimer lamps are unwieldy, making them suitable only for stationary fittings. They are also a legacy technology.
That’s because over the past decade or so, we have gradually seen incandescent and fluorescent lighting supplanted by that based on LEDs, which are cheaper, more efficient, more tunable, safer (because they are lower voltage) and longer lasting. This solid-state revolution has been brought about largely thanks to Isamu Akasaki, Hiroshi Amano and Shuji Nakamura, who in the early 1990s developed the first blue LEDs, for which they were later awarded the 2014 Nobel Prize for Physics. The output of blue LEDs can be easily converted to white by adding a phosphorescent layer, making them convenient for all sorts of lighting applications, including the backlights of flat-screen monitors.
This is why Ironside sees LEDs as the most convenient source of far-UVC. “If we did produce a far-UVC LED that was proven to be safe [for humans] then I think it would make a big difference,” he says. “All you have to do is envisage what it would be like if there was an infection-control device available on every mobile phone now – the device could be used to sterilize surfaces and hands.”
“There will always be a period after a new pathogen has evolved, and before a vaccine is available, when the first line of defence is infection control,” he continues. “A far-UVC light LED will be a major component… It could also revolutionize personal-protection equipment for health workers.”
In principle, there is no reason why LEDs cannot be manufactured to emit at almost any wavelength, by adjusting the alloys of the semiconductors used
In principle, there is no reason why LEDs cannot be manufactured to emit at almost any wavelength, by adjusting the alloys of the semiconductors used. Gallium nitride (GaN), for example, which forms the basis of most commercial LEDs, has a band gap of about 3.4 eV, corresponding to a visible violet emission of wavelength 360 nm. The band gap of aluminium nitride (AlN), meanwhile, is about 6.4 eV, corresponding to a natural emission very deep in the UVC, at 210 nm. As a result, Al-GaN LEDs emit light at wavelengths somewhere in between, depending roughly on the ratio of aluminium to gallium.
Far-UVC LEDs based on such alloys have already been demonstrated in the lab. Since 2007, for instance, Hideki Hirayama at the Riken research institute in Saitama, Japan, and colleagues have been creating Al-GaN LEDs with emission wavelengths down to 222 nm (Electr. Commun. Jpn 10.1002/ecj.11667). Meanwhile, with an emission at 210 nm, the shortest-wavelength UVC LED was demonstrated in 2006 by Yoshitaka Taniyasu and colleagues at the NTT Basic Research Laboratories in Atsugi, Japan, based on pure AlN (Nature 441 325).
Unfortunately, these lab devices have an efficiency of barely a few per cent – well below the 20–40% needed for practical use – which means that none of them has ever been commercialized. Despite LEDs becoming available at shorter and shorter wavelengths – with Nitride Semiconductors in Japan even offering one at 275 nm – most commercially available UV LEDs emit at UVA wavelengths of about 350 nm, where they find applications in the curing of adhesives and ink-jet printing.
Inside an LED

A light-emitting diode (LED) essentially consists of an “active” layer of semiconducting material sandwiched between negatively doped (n-type) and positively doped (p-type) semiconductors. When a voltage is applied to the junction, electrons from the n-type material move into the conduction band of the active layer, while holes from the p-type semiconductor are injected into the valence band. Light emission takes place when electrons at the bottom of the conduction band spontaneously recombine with holes in the top of the valence band in what are called quantum wells. The energy difference between the bands, known as the band gap, dictates the wavelength of the released photons.
Out of the darkness
Rob Harper, the GaN programme manager at Compound Semiconductor Centre (CSC) – a joint venture between the semiconductor wafer manufacturer IQE and Cardiff University in the UK – explains that one of the problems in reliably manufacturing efficient far-UVC LEDs is the doping of Al-GaN semiconductors with metals such as indium to make them slightly positive or “p-type”. When the dopants are added, they tend to leak into the light-emitting region of the LED, stifling light emission, he says. And when grown via the industry-standard epitaxy technique of metal organic chemical vapour deposition, the high aluminium content itself degrades the crystalline structure. “Higher than usual epitaxial growth temperatures [can be used to] alleviate this, but result in increased incorporation of unintended impurities in the active region,” he says. “[These] also quench light generation and result in very low efficiency.” Another potential far-UVC alloy, magnesium zinc oxide (Mg-ZnO), suffers similar problems, he adds.
Still, Harper understands why the challenge is so important. “The current COVID-19 outbreak has painfully illustrated the need for new approaches to cost-effective, rapidly deployed, large-area disinfection techniques such as UVC irradiation,” he says. “Any research approach that shows potential to realize new practical p-doping techniques merits investigation.”
Harper did not disclose whether CSC plans to work on far-UVC LEDs. However, one researcher who has responded to Ironside’s call to arms is Tony Kelly, a former colleague and a “commercial turned academic” researcher in applied optoelectronics at the University of Glasgow in the UK. Within two days of being contacted by Physics World about the topic, he had already investigated potential funding avenues and was in the process of seeking collaborators for a far-UVC LED research project. “Often Charlie is right about things, and I think he’s right about this,” he says.
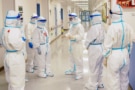
COVID-19: how physics is helping the fight against the pandemic
Like Harper, Kelly can foresee problems in making efficient devices. The shift to such emission from where UV LEDs are currently, he says, “could be a lot harder than it looks”. Still, he has reasons to be positive. Many funding agencies are urgently seeking projects whose results could mitigate the effects of the COVID-19 pandemic, and he expects them to be fast-tracked. UK Research and Innovation, for example, currently has an open-ended call for proposals of any financial scale related to COVID-19 that could deliver results in 18 months. “As with everything, the potential impact is driven by current affairs,” says Kelly. He jokes that, ironically, the biggest delay could be getting back into his lab to build prototypes. Like almost every other academic institution, Glasgow University is currently [as of early May] closed to all but essential staff and researchers. Those conducting science relevant to COVID-19 fit into this category; nevertheless, the practicalities of running a clean room, introducing social-distancing measures, and deciding who should be allowed in it, are issues that Kelly will have to resolve with his university’s administrators.
Although the investment in manufacturing tools and production lines for new semiconductor devices can be eye-watering, often running into billions, Kelly thinks this scale of investment may not ultimately be necessary for Al-GaN LEDs, as GaN is already an established commercial material. Instead, it will be a matter of adapting the fabrication plants that already exist. “If we find a design that works in a year, we could start raising finance to get on with things,” Kelly says.
Meanwhile, Ironside himself is not shirking the challenge. Although most of his research to date has focused on near- to mid-infrared LEDs, he is hoping to obtain joint funding with an industrial partner to explore the far-UVC potential of Mg-ZnO LEDs. He believes that success will be due to innovative physics combined with manufacturing expertise.
Naturally, this success is not guaranteed. But with governments across the world spending billions to keep their economies afloat, the incentive to find ways to avoid future chaos is as much commercial as humanitarian, and Ironside wants as many of his fellow researchers as possible to get involved. “As soon as I heard about Brenner’s work on the far-UVC, I thought it was an idea that was really worth pursuing,” he says. “I think the community should be aware of it.”
Analysis: Despite bad press linked to Donald Trump, UV light could combat future pandemics
By Matin Durrani, editor-in-chief, Physics World
Many of Donald Trump’s supporters – and surely all his detractors – would agree that he’s said and Tweeted some pretty bizarre and controversial things during his time as US president. But even by his standards, Trump’s utterances in a press conference at the end of April were off the scale. Speaking to reporters in the White House, the 45th US president mused on whether ultraviolet (UV) light could tackle the spread of the virus behind the COVID-19 pandemic.
“Supposing we hit the body with a tremendous…ultraviolet or just very powerful light. And then supposing you brought the light inside the body, either through the skin or in some other way. Sounds interesting. The whole concept of the light, the way it kills the virus…that’s pretty powerful.” Unfortunately, while UV can kill viruses, certain frequencies are incredibly dangerous to humans. To make matters worse, Trump then wondered out loud if COVID-19 could be treated by injecting disinfectant into the body.
Trump is no scientist, despite once telling the Boston Globe that he shared the same “very good genetics” as his uncle John Trump, who was a physicist at the Massachusetts Institute of Technology for nearly five decades. Indeed, the president’s comments forced two US-based UV trade organizations to release a joint statement to remind the public that there is no accepted safe means of exposing the human body to UV to kill viruses. Dettol manufacturer Reckitt Benckiser also had to reiterate that “under no circumstance should our disinfectant products be administered into the human body”.
It’s long been known that UV kills viruses by damaging their DNA – indeed, UV light from fluorescent tubes is already used in hospitals to sterilize equipment and surfaces. Trouble is, the light from these tubes – 250 nm, right in the middle of the UVC band – is believed to damage cellular DNA and sometimes trigger cancer. People are therefore not permitted anywhere near UVC germicidal lamps without suitable protection.
However, there has been recent research (see above) suggesting that a small band of far-UV light (at roughly 220 nm) can damage viruses yet still be safe for humans. That raises the prospect of far-UV being used to kill viruses in hospitals, trains, shops and other places even where people are present. There’s a long way to go before this notion becomes reality. Apart from fully corroborating that far-UV is safe, we’d also need a simpler way to create this light than the large, unwieldy “excimer lamps” that are currently used.
That’s why some physicists are calling for research into far-UV LEDs, which would be much smaller and could turn everyone’s mobile phone into a virus-buster. It would be a shame if Trump’s ramblings on UV ended up discrediting a potentially promising avenue of physics-based research that could help us avoid another pandemic.