
Flocks of birds, schools of fish and swarms of insects are all examples of “active matter” – systems of particles that move on their own without recourse to external forces. Scientists have long sought to replicate such behaviour in the laboratory, with the aim of producing synthetic materials that mimic biological functionalities such as self-healing or cell mobility and division. A team of researchers in California, US, has now moved closer to this goal by obtaining the first detailed, three-dimensional views of a type of active matter known as nematic liquid crystals.
The team made their observations using a technique called light-sheet microscopy, which was originally developed to visualize how living organisms acquire their shape and architecture. According to co-team leader Zvonimir Dogic of the University of California, Santa Barbara, these observations represent the first step towards real-world applications for active crystals, as previous studies had mostly focused on simpler two-dimensional systems.
Non-equilibrium state
Nematic liquid crystals get their name from the Greek word nema, meaning “thread” – a reference to their characteristic thread-like topological defects. These defects are called disclination lines, and they can be made of elongated molecules or colloidal particles. Within a given area, they will on average point in the same direction, along a localized preferential axis known as the director.
In ordinary, passive, nematics, homogenously aligned states are favoured over deformations that cause the material to bend. In active nematics, however, the situation is different, because the active components provide energy on the microscale throughout the structure. This energy drives internal stresses that distort the fluid, with the result that even the smallest deformations can amplify exponentially.
This non-equilibrium state cannot be described in the framework of conventional thermodynamics, and it leads to unsteady, active turbulent flows in the bulk of the material. In contrast to turbulence in normal fluids, the chaotic flow in active nematics does not contain regular patterns and can give rise to large-scale coherent motion, producing emergent structures, such as phase boundaries and topological defects, in which local order has broken down.
Bending instabilities and bulk flows
In their new work, Dogic and his colleagues, together with a team led by Daniel Beller from the University of California, Merced, made their active nematic fluids from bacteriophage virus particles. The rigid rods of the virus provide the nematic liquid crystal phase at room temperature.
Building on earlier work in Dogic’s lab, the teams dispersed two basic components of biological cells – microtubules and kinesin motors – into the nematic phase to create their active material. Microtubules are filaments of long, semi-flexible protein assemblies, while kinesin is a protein that can convert chemical energy (in the form of adenosine triphosphate, or ATP) to mechanical energy.
When fuelled with ATP, the molecular motors elongated the microtubule bundles, which in turn induced active stresses that created bending instabilities and bulk flows throughout the entire sample, including the nematic phase.
Tracking the motion of defects in real time
To study this behaviour, the team turned to light-sheet microscopy. In this technique, a micron-thin sheet of light is used to image a sample of molecules labelled with a fluorescent marker. By rapidly scanning the sheet along the z-direction of the sample, the researchers could image their active nematic crystals with molecular-scale resolution and track the motion of defects in real time. This approach provides a quantitative measure of the local director field over the entire 3D volume of the active material.
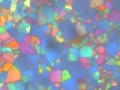
The secrets of the blue fog
The researchers found that their nematics contain both disclination lines and loops, including Möbius-strip-like objects that can nucleate, shrink, open and merge to form spatially extended structures. These disclinations can be thought of as rubber-band-like loops that are continuously being pulled and stretched by internal forces, they say.
To understand how the dynamics of these loops depends on the material’s local topological structure, Dogic says that he and his colleagues will need to develop visualization techniques with improved temporal resolution as well as new methods to isolate single loops. “We would also like to gain a detailed appreciation of how an isolated loop appears in a perfectly aligned material,” he tells Physics World.
The new work, which is detailed in Science, is an important example of how to make and study 3D active crystals, Dogic adds. In a related Perspectives article, Dennis Bartolo, a researcher at the University of Lyon, France, who was not involved in the work, called it “a formidable experimental platform” for future observations of these complex materials.