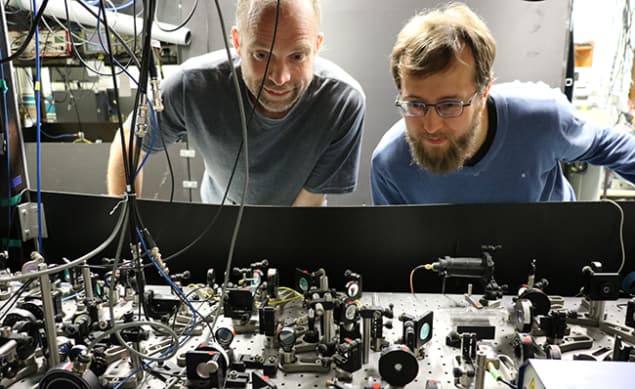
Physicists in New Zealand have used optical tweezers to combine three atoms, with two of the atoms forming a molecule in the presence of the third. They were able to measure the rate at which this “three-body recombination” occurs and found it to be much lower than had been expected. The technique could be used to provide important information about how atoms combine to form molecules.
In atomic physics three is a crowd because it is notoriously difficult to calculate how three or more atoms will interact with each other to form a molecule. “Fundamentally, we think that, if we write up the equations of motion from quantum mechanics, these will describe our system,” explains atomic physicist Mikkel Andersen at the University of Otago in Dunedin. “But you can only do that and get an exact solution for a very, very simple system. The question then often becomes finding out, under different conditions, what’s important and what we can throw out of the equations.”
The question is not just of academic interest, as everything around us results from atoms combining. Previous researchers have studied atomic combination using Bose–Einstein condensates, but these contain many atoms, which makes it difficult to disentangle various effects. “We’re trying to develop the capability to build small quantum systems such as molecules atom by atom,” explains Andersen.
Scattered photons
In the new work, Andersen and colleagues at Otago and Massey University in Auckland cooled three atoms of rubidium to 17.8 μK in three separate optical traps positioned about 4.5 μm apart. They then carefully merged the three traps, allowing the atoms to interact. After about a second, the researchers irradiated the combined trap with light, counted the number of photons it scattered and inferred from this how many atoms it contained.
By repeating the experiment multiple times with the same time interval between merging and measurement, the researchers measured the probability of the trap containing various numbers of atoms after that time interval. By varying the time interval, the researchers measured how these probabilities evolved in time.
The results provide the first-ever observation of three-body collisions and combinations at an atom-by-atom level. Andersen explains that the results provide important information about how molecules form: “If you put two atoms in an optical tweezer they don’t usually form a molecule by themselves because something else needs to take away the binding energy But if you put in three, two of them can form a molecule, with and the third taking away the binding energy.”
Lower rate
The researchers were surprised that the rate of this “three-body recombination” was more than ten times lower than theoretical predictions and previous experimental work in Bose–Einstein condensates had suggested. Indeed, Andersen was so perplexed by the results that he had an independent group of people repeat the measurements. “I was convinced it could not be true!” he recalls.
Since describing the research in Physical Review Letters, he says, “We have been contacted by a number of people from the scientific community with suggestions as to what could be going on so, in the next year, we will be conducting a number of experiments to verify whether or not these suggestions are actually true.”

Two new surprises for three-body physics
Cold-atom experimentalist Hans-Christoph Nägerl of the University of Innsbruck in Austria, who works with Bose–Einstein condensates, is impressed by the work. “I think this bottom-up approach to looking at few-body processes really has a future,” he says. “I think we’ll see a lot of results with similar systems.”
He is less convinced by the team’s conclusions regarding the recombination rate, however. “First of all, the temperature is not really ultracold, so I’m not too surprised that the rates are different from the zero-temperature expectations,” he says. “The second point – which they raise – is the role of confinement. A few years back my group published a paper – which they don’t cite – looking at what happens to correlations in confined dimensions. We only looked at the repulsive case, but the evidence clearly showed that confinement strongly modifies the three-body correlations.” His conclusion is that “it’s a very nice addition to what’s been done previously. Will one see new physics? It’s hard for me to judge.”