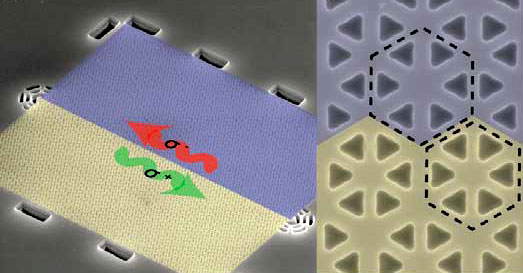
Single photons have been emitted into topological edge states by physicists in the US. The research provides a direct link between quantum optics and topological photonics, and could prove important for a wide range of applications, including quantum communication and quantum computing.
Topological insulators are electrical insulators in the bulk, but electrons at the edge of such a material can travel without scattering in one direction that depends on their spin polarization. Similar topological behaviour has also been seen in a wide variety of other systems such as mechanical vibrations and light. The possibility of moving single photons around without scattering has attracted particular interest for potential applications in quantum-information processing. However, quantum optician Mohammad Hafezi of the Joint Quantum Institute at the University of Maryland, College Park explains that although topological photonics is an inherently quantum phenomenon, it has only ever been demonstrated with classical light.
Honeycomb symmetry
Now, Maryland researchers led by Hafezi and Edo Waks have created a heterostructure containing two adjoining periodic optical nanostructures called photonic crystals, each comprising distorted gallium arsenide honeycomb lattices. The perfectly regular lattice transmits photons of any frequency: “You can think of it basically as graphene,” says Hafezi. “Any system that has this honeycomb symmetry has this Dirac cone that does not have any band gap.” Distorting the lattice structure, however, opens up an optical band gap. In the researchers’ hereterostructure, photons around 950 nm wavelength would not propagate.
In one photonic crystal the researchers moved the triangular holes of each hexagon closer to the hexagon’s centre. In the other they moved the holes further apart. The optical band gaps created by these two distortions are such that the energy band that sits above the energy gap in one photonic crystal sits underneath it in the other, and vice versa. At the edge where the two photonic crystals meet, therefore, the two bands have to touch and cross over. This produces an edge state with an energy that lies in the middle of each photonic crystal’s band gap. Photons with this energy can therefore travel between the two photonic crystals but never scatter into the bulk. Symmetry considerations mean that photons with one circular polarization travel in one direction, whereas photons with the opposite circular polarization travel the opposite way.
Embedded quantum dots
The researchers embedded indium-arsenide quantum dots inside the heterostructure. At first they used a relatively high-power laser, exciting the quantum dots to emit broadband light. When they focused the laser on one side of the edge, they found that light in the bulk band gap travelled straight through without scattering, whereas light at other frequencies scattered into the bulk.
For their next experiment the researchers turned down their laser to 10 nW and focused it on individual quantum dots, exciting them to emit photons one at a time. To work out if photons with opposite polarizations would travel in opposite directions, the researchers applied an external magnetic field to separate the energies of the two polarization states. They found that, as predicted, photons detected at one end of the edge state had a higher energy than those detected at the other end. Finally, the researchers introduced a 60° bend into the edge: photons in the edge state tracked this perfectly without reflecting or scattering into the bulk. “Now our photons can, in principle, strongly interact with each other,” says Hafezi. “So now all these ideas of many-body physics and quantum-information processing can be considered in the context of topological photonics.”
“It’s definitely exciting,” says Peter Lodahl of the Niels Bohr Institute at the University of Copenhagen in Denmark. “It’s a major experimental step forward to demonstrate true topological phenomena in a quantum regime.” His own group first demonstrated that the direction of photons from single quantum emitters in waveguides can depend on their spin. “It’s too early to tell to what extent this topological addition gives you practical advantages,” he says, “but it’s a really exciting thing to investigate further.”
‘Big step forward’
“This [work] is a big step forward in the implementation of new optical properties in materials,” agrees Alberto Amo of the University of Lille. “The next step would be to go further with these ideas of quantum optics in topologically protected circuits and connect two or more single-photon emitters such that they can start to interact. When you have that, you can implement quantum gates and other quantum optics protocols.”
The research is described in Science.