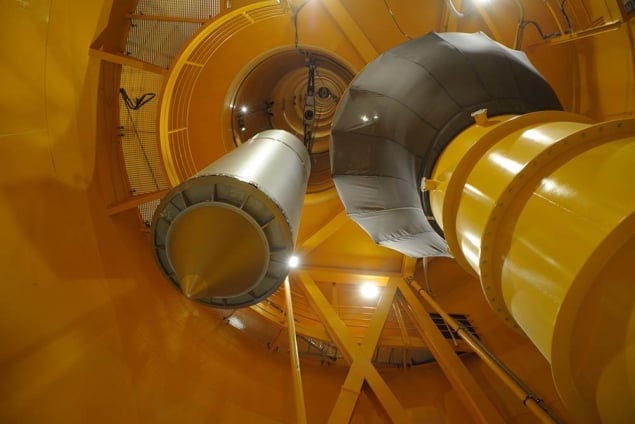
The atmosphere on Mars doesn’t support human life, so if humanity is going to travel to – and survive – on the red planet, we’ll need to pack our space rockets with everything needed to support life. That will be a heavy load, so it’s no surprise that space agencies are looking to develop life-support technologies and renewable fuel production that are lightweight and regenerative.
This makes photoelectrochemical cells, which convert solar energy to produce oxygen while simultaneously releasing hydrogen for fuel, an attractive option. Now, solar-fuel researchers in California and Germany have demonstrated a semiconductor half-cell that, unlike conventional designs, efficiently releases hydrogen in microgravity conditions.
“We wanted to figure out if we can actually do photochemistry in microgravity and produce fuels,” says first author Katharina Brinkert from the California Institute of Technology. But testing and designing a cell that’s capable of working under zero-gravity conditions proved quite the challenge.
Dropping into microgravity
Experiments in zero-gravity aren’t easily conducted here on Earth. But at the Center of Applied Space Technology and Microgravity (ZARM) in Bremen, Germany, there is a specially designed drop tower that enables short experiments under weightless conditions.
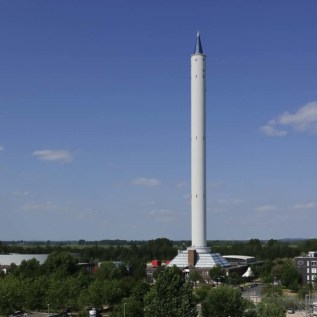
In the experiments at ZARM, all the equipment must be installed into a single capsule that is launched up the 120 m tower by a pneumatic piston, reaching 168 km/h. When the capsule falls it experiences weightlessness for 9.3 seconds, and it’s in that fall-time that experiments must be conducted. “We weren’t sure the experiment would work because we only had 9.3 seconds of microgravity to perform difficult electrochemistry,” says Brinkert.
Brinkert says that the research team relied on the expertise of the scientists at ZARM to set up and automate the experiment. And to make it easier, the team chose to test the simplest half of the photoelectrochemical cell, where hydrogen is released at the photocathode.
The researchers already knew that the efficiency of the electrodes in traditional solar-fuel cells falls in microgravity. “In microgravity conditions you have an absence of buoyancy and that makes the gas bubbles produced stick to the electrode surface,” says Brinkert. These hydrogen bubbles gather together to coat electrodes in a “froth layer” that increases resistance and reduces current and voltage through the electrode.
As expected, the team’s traditionally designed, indium-phosphide photocathodes with flat rhodium deposits experienced up to a 70% reduction in circuit voltage, with froth formation evident on camera footage. To prevent the formation of the froth layer, the California-based solar-fuel scientists sought help from Michael Giersig at the Freie University in Berlin, who is an expert at creating nanostructured surfaces that can alter the properties of component materials. While nanostructuring catalysts is nothing new in the solar-fuel community, Giersig specializes in shadow nanosphere lithography (SNL), which has not previously been used with solar-fuel cells in microgravity.
The nanostructured fuel cells were composed of the same indium phosphide, but the rhodium was deposited using SNL. Employing latex spheres as a type of template, the scientists were able to form the rhodium into 3D hexagonal nanostructures.
Tests in the drop tower revealed that the nanostructured cells performed much better than the traditional cells in microgravity, with only a 25% drop in voltage. Experiments in terrestrial conditions yielded the same efficiencies between catalyst designs, confirming that the difference in voltage generated in microgravity was related to surface topology.
Images of bubble formation in microgravity combined with theoretical analyses helped the researchers to understand how nanostructuring improved performance. “Our theory is that the bubbles are produced along the tips (of the 3D structures) and that these tips are so small that this limits the gas bubble growth,” says Brinkert.
Completing the cell
The researcher’s findings suggest a new design principle that could help realize simple and lightweight life-support systems for future space travel. Shaowei Chen, a professor of electrochemistry at the University of California, Santa Cruz, also thinks this technique could improve the performance of terrestrial water splitting technologies.
Brinkert and colleagues are now keen to advance their studies to look at the other half of the cell, which releases oxygen for life support. “We’d like to have two half cells working together, splitting water at the photoanode and feeding electrons to the photocathode to reduce the species you want to produce for fuel,” she explains. “Ultimately we’d like to take such a device up to the international space station and do experiments there.”
The research is described in Nature Communications.