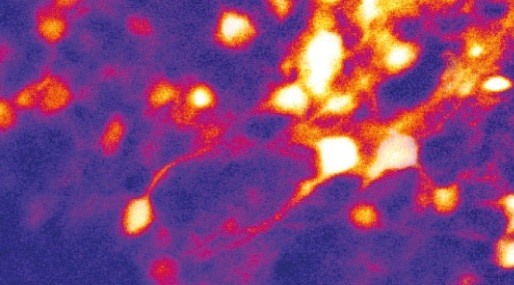

Arguably the biggest scientific challenge of the century is to delve into how the brain works – what stimulus activates which neuron(s) and how does that affect behaviour and thinking? There are a lot of different technologies with brain mapping functionality, but none have the resolution needed to track underlying cellular and molecular patterns in the brain.
Scientists want to combine the temporal resolution of electrophysiology recordings with the spatial resolution acquired with two-photon imaging of neuronal calcium fluctuations. But this requires fabrication of a special electrode that possesses good electrical function for electrophysiology, whilst allowing light to pass through for two-photon imaging.
A number of different materials have been trialled for use in transparent electrodes, but when scaled to measure single neuronal activity, the electrodes all had poor electrical performance. Signal-to-noise ratio suffers, because at the micrometre scale, complex resistance leads to high impedance and corresponding large noise.
Over the past two years, Hui Fang‘s group at Northeastern University produced a new type of transparent microelectrode material. It was fabricated from traditional electrode materials with nanoscale “holes” forming a lace-like, nanomesh structure that imparts macroscale transparency.
Fang has now developed his material into a fully functioning microelectrode array and collaborated with neurobiologist Michela Fagiolini from Boston Children’s Hospital to validate electrode functionality in the visual cortex of live mice (Science Advances 4 eaat0626).
“We’ve bridged two major brain mapping modalities,” says Fang, pointing out that the mapping this microelectrode achieves in small animals could be correlated to humans, with potentially “enormous” impact on understanding the brain.
Nano-meshing
To turn the metal nanomeshes into high-performance electrodes, the bioengineers applied a low-impedance coating of poly(3,4-ethylenedioxythiophene) polystyrene sulfonate, creating a 25 nm-thick gold/85 nm-thick coating bilayer. However, this was a fiddly task, as the lace-like holes in the coating needed to perfectly align with those in the metal nanomesh to prevent blocking the nanoholes and thereby light’s passage through the electrode. Fang credits the students in his team for the diligent work that eventually led to a coating that faithfully protected the transparent nanomesh structure.
Scalability was demonstrated, with 130 kW impedance at 1 kHz for each of the 20 µm diameter channels, a performance comparable to the best non-transparent electrode arrays. “The high yield we found from bench testing was really remarkable,” says Fang. Mechanical bending and long-term soaking tests also showed that the electrodes were physically and chemically robust and flexible.
Before in vivo testing, the team needed to remove artefacts in electrophysiology recordings known to be induced by neuronal stimulation. They established a collaboration with bioengineers at the University of California, Los Angeles, who used their advanced artefact rejection and wireless system to remove artefacts from recordings.
Live neurons
Fangolini’s group at Boston Children’s Hospital took the promising microelectrode array and tested its capabilities in live mice.

The transparent microelectrodes were implanted with cranial windows into the skulls of adult mice, and a headbar was fitted to enable head restraint when imaging. After surgery, a calcium indicator expressing virus was injected into the visual cortex.
Twenty days after surgery, the team applied visual stimuli and made concurrent electrophysiology readings alongside two-photon imaging of neurons in layer 2/3 of the visual cortex.
Electrophysiology readings achieved high yields with stable low impedance throughout, whilst high transparency enabled good two-photon image quality. “We validated that electrophysiology matches calcium imaging,” says Fang.
But Fang also points out that the calcium dynamics limited the two-photon imaging to low frequencies. “At low frequency level the data matched, but at high frequencies we can only rely on electrophysiology,” he notes.
Mapping neuronal activity
Fang’s group is now working on new transparent electrode arrays that have hundreds of channels. However, advanced algorithms need to be developed to accurately map each signal detected to individual neurons.
“Our next step is to take the good spatial resolution from calcium imaging, and the good temporal resolution from electrophysiology and fuse the data together to provide a complete picture,” explains Fang.
The group hope that, despite the fact that most optical methods cannot be ethically performed on humans, this work will lead to insights into human neurobiology. “If we can better understand how optical mapping and electrophysiology correlates together, we can better infer how the human brain is functioning from the human electrophysiology data,” says Fang.