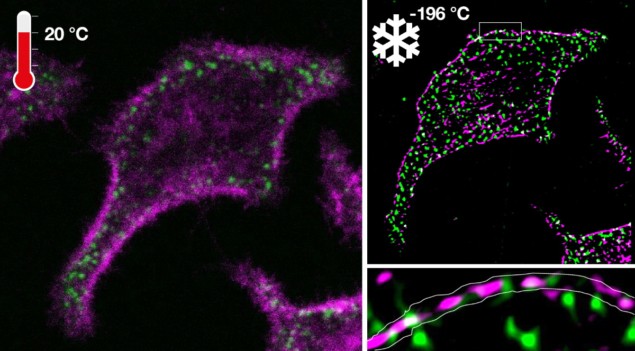
The energized state of living matter leads to the dynamic behaviour of billions of interacting nanometre-sized biomolecules, and the spatial–temporal patterns resulting from this collective behaviour give rise to cellular functionalities that manifest at the micrometre scale.
Advances in imaging technology have allowed us to capture pattern-forming biomolecular dynamics using fluorescent probes to achieve mind-blowing spatial and temporal resolution. Fluorescence microscopy, for example, can resolve patterns down to the nanoscale via techniques such as single-molecule localization microscopy, nanoscopy with minimal photon flux and stimulated emission depletion nanoscopy.
The total number of collected photons determines the signal-to-noise ratio and the achievable spatial or spectroscopic resolution. A maximally attainable photon flux from the optical probes therefore sets a lower limit to the time needed to collect enough photons to attain a particular resolution. During this photon collection time, however, passive or active motion of molecules results in blur, thereby limiting the resolution of fluorescent structures. This fundamental limit, set by the photophysical properties of the fluorophores, cannot be overcome by better detectors or stronger illumination.
Another problem is that the interaction of light with the fluorescent probes produces toxic radicals, which not only destroy molecular processes and eventually kill the cells, but also destroy the fluorescent molecule itself, limiting the number of photons that can be collected.
One way to overcome motional blur is to arrest the very dynamics of biomolecular processes, thus enabling any photon collection time. This can, in principle, be achieved by chemical fixation of cells. However, chemical fixation processes that change the sample by cross-linking or denaturation take a long time and disintegrate the living patterns into a dead state. The dynamic out-of-equilibrium molecular patterns of living matter can therefore not be captured, and photobleaching still limits photon collection.
To bypass chemical fixation and halt photoreactivity, a workaround is to physically fix the cells at a particular instant in time by extremely rapid cooling to below –136 °C, the temperature at which water becomes a disordered solid. This ultrarapid cooling is essential for two reasons: to maintain an out-of-equilibrium water state that prevents mechanical damage by ice crystal formation (which occurs between 0°C and –136 °C); and to avoid disintegration of the energized microscopic biomolecular patterns to the dead state.
Researchers in the Department of Systemic Cell Biology at the Max Planck Institute of Molecular Physiology, headed by Philippe Bastiaens, have developed ultrarapid cryo-arrest microscopy that circumvents all the above-mentioned limitations. The novel approach, described in Science Advances, cools living cells directly on a microscope at speeds of up to 200,000 °C/s – enabling the preservation and multi-scale observation of cellular and molecular patterns in their native state at the time of arrest.
Working principle
Jan Huebinger and colleagues in Bastiaens’ research group developed a technology for ultrarapid cooling integrated with a multimodal fluorescence microscope, enabling virtually instant cryo-arrest of biological processes. To perform the cryo-arrest, cells are adhered to a diamond heat exchanger that can be rapidly cooled by a burst of pressurized liquid nitrogen (at –196 °C). In other words, the researchers adhered living cells grown on a glass coverslip to one of the best heat conductors on Earth. On the opposite side of the coverslip, a microscope objective enables them to observe the cells during the cold-arrest.
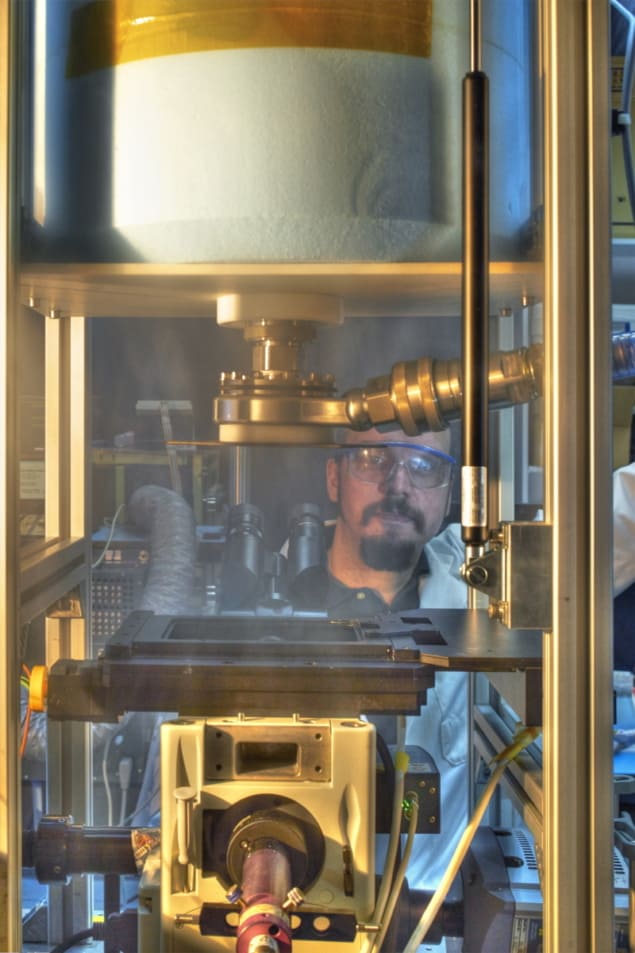
To create the high-pressure burst of cold, the researchers injected liquid nitrogen and pressurized gaseous helium into a vessel. An electronically triggered valve then releases a burst of cold from this vessel onto the diamond. A pressure-controlled valve, which detects the drop in pressure during the cold shot, ensures a subsequent constant flow of liquid nitrogen to maintain the sample temperature close to −196 °C.
The high-pressure burst combined with the remarkable heat conductance of diamond enabled the researchers to achieve extremely high cooling rates and arrest living cells in their native state during microscopic observation. This novel approach allowed them to take long-exposure snapshots of native molecular patterns and reaction states in cells, at scales that are otherwise invisible.
The researchers assessed their novel ultrarapid cryo-arrest development by investigating the underlying molecular organization of an oncoprotein and a tumour-suppressor protein. The absence of motional blur and enhanced photostability of fluorescent markers revealed a previously unknown co-organization of onco- and tumour-suppressor proteins into nanoscale clusters along the plasma membrane. This enabled the resolution of a missing link in how information processing by cancer-related molecules in biomolecular networks can switch from a dynamically silenced to a transient active signalling state.
“This is an enabling step for fluorescence microscopy, especially the combination of super-resolution microscopy and microspectroscopy that allows the mapping of molecular reactions in cells at multiple scales. It will change the way we observe molecular organization and reaction patterns in cells and therefore provide more insight into the self-organizing information-processing capabilities of living matter,” says Bastiaens.