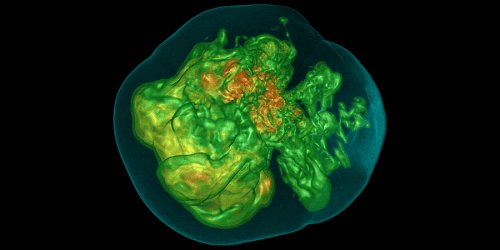
Permanent distortions in space–time caused by the passage of gravitational waves could be detectable from Earth. Known as “gravitational memory”, such distortions are predicted to occur most prominently when the core of a supernova collapses. Observing them could therefore provide a window into the death of massive stars and the creation of black holes, but there’s a catch: the supernova might have to happen in our own galaxy.
Physicists have been detecting gravitational waves from colliding stellar-mass black holes and neutron stars for almost a decade now, and theory predicts that core-collapse supernovae should also produce them. The difference is that unlike collisions, supernovae tend to be lopsided – they don’t explode outwards equally in all directions. It is this asymmetry – in both the emission of neutrinos from the collapsing core and the motion of the blast wave itself – that produces the gravitational-wave memory effect.
“The memory is the result of the lowest frequency aspects of these motions,” explains Colter Richardson, a PhD student at the University of Tennessee in Knoxville, US and co-lead author (with Haakon Andresen of Sweden’s Oskar Klein Centre) of a Physical Review Letters paper describing how gravitational-wave memory detection might work on Earth.
Filtering out seismic noise
Previously, many physicists assumed it wouldn’t be possible to detect the memory effect from Earth. This is because it manifests at frequencies below 10 Hz, where noise from seismic events tends to swamp detectors. Indeed, Harvard astrophysicist Kiranjyot Gill argues that detecting gravitational memory “would require exceptional sensitivity in the millihertz range to separate it from background noise and other astrophysical signals” – a sensitivity that she says Earth-based detectors simply don’t have.
Anthony Mezzacappa, Richardson’s supervisor at Tennessee, counters this by saying that while the memory signal itself cannot be detected, the ramp-up to it can. “The signal ramp-up corresponds to a frequency of 20–30 Hz, which is well above 10 Hz, below which the detector response needs to be better characterized for what we can detect on Earth, before dropping down to virtually 0 Hz where the final memory amplitude is achieved,” he tells Physics World.
The key, Mezzacappa explains, is a “matched filter” technique in which templates of what the ramp-up should look like are matched to the signal to pick it out from low-frequency background noise. Using this technique, the team’s simulations show that it should be possible for Earth-based gravitational-wave detectors such as LIGO to detect the ramp-up even though the actual deformation effect would be tiny – around 10-16 cm “scaled to the size of a LIGO detector arm”, Richardson says.
The snag is that for the ramp-up to be detectable, the simulations suggest the supernova would need to be close – probably within 10 kiloparsecs (32,615 light-years) of Earth. That would place it within our own galaxy, and galactic supernovae are not exactly common. The last to be observed in real time was spotted by Johannes Kepler in 1604; though there have been others since, we’ve only identified their remnants after the fact.
Going to the Moon
Mezzacappa and colleagues are optimistic that multimessenger astronomy techniques such as gravitational-wave and neutrino detectors will help astronomers identify future Milky Way supernovae as they happen, even if cosmic dust (for example) hides their light for optical observers.
Gill, however, prefers to look towards the future. In a paper under revision at Astrophysical Journal Letters, and currently available as a preprint, she cites two proposals for detectors on the Moon that could transform gravitational-wave physics and extend the range at which gravitational memory signals can be detected.
The first, called the Lunar Gravitational Wave Antenna, would use inertial sensors to detect the Moon shaking as gravitational waves ripple through it. The other, known as the Laser Interferometer Lunar Antenna, would be like a giant, triangular version of LIGO with arms spanning tens of kilometres open to space. Both are distinct from the European Space Agency’s Laser Interferometer Space Antenna, which is due for launch in the 2030s, but is optimized to detect gravitational waves from supermassive black holes rather than supernovae.
“Lunar-based detectors or future space-based observatories beyond LISA would overcome the terrestrial limitations,” Gill argues. Such detectors, she adds, could register a memory effect from supernovae tens or even hundreds of millions of light-years away. This huge volume of space would encompass many galaxies, making the detection of gravitational waves from core-collapse supernovae almost routine.
The memory of something far away
In response, Richardson points out that his team’s filtering method could also work at longer ranges – up to approximately 10 million light-years, encompassing our own Local Group of galaxies and several others – in certain circumstances. If a massive star is spinning very quickly, or it has an exceptionally strong magnetic field, its eventual supernova explosion will be highly collimated and almost jet-like, boosting the amplitude of the memory effect. “If the amplitude is significantly larger, then the detection distance is also significantly larger,” he says.
Whatever technologies are involved, both groups agree that detecting gravitational-wave memory is important. It might, for example, tell us whether a supernova has left behind a neutron star or a black hole, which would be valuable because the reasons one forms and not the other remain a source of debate among astrophysicists.
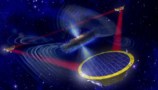
European Space Agency gives construction go-ahead for LISA gravitational-wave mission
“By complementing other multimessenger observations in the electromagnetic spectrum and neutrinos, gravitational-wave memory detection would provide unparalleled insights into the complex interplay of forces in core-collapse supernovae,” Gill says.
Richardson agrees that a detection would be huge and hopes that his work and that of others “motivates new investigations into the low-frequency region of gravitational-wave astronomy”.