Astrophysicists Maura McLaughlin and Duncan Lorimer talk to Sidney Perkowitz about everything from their pioneering pulsar research to building a life together and eventually winning the 2023 Shaw Prize in Astronomy

Most physicists dream of making new discoveries that expand what we know about the universe, but they know that such breakthroughs are extremely rare. It’s even more surprising for a scientist to make a great discovery with someone who is not just a colleague, but also their life partner. The best-known husband-and-wife couples in physics are the Curies, Marie and Pierre; as well as their daughter, Irène Joliot-Curie and her husband Frédéric Joliot-Curie. Each couple won a Nobel prize, in 1903 and 1935 respectively, for early work on radioactivity.
Joining the ranks of these pioneering physicists are contemporary married couple Maura McLaughlin and Duncan Lorimer, who last year were two of three laureates awarded the $1.2m Shaw Prize in Astronomy (see box below) for their breakthroughs in radio astronomy. Together with astrophysicist Matthew Bailes, director of the Australian Research Council Centre of Excellence for Gravitational Wave Discovery, McLaughlin and Lorimer won the prize for their 2007 discovery of fast radio bursts (FRBs) – powerful but short-lived pulses of radio waves from distant cosmological sources. Since their discovery, several thousand of these mysterious cosmic flashes, which last for milliseconds, have been spotted.
Over the years, McLaughlin and Lorimer’s journeys – through academia and their personal life – have been inherently entwined and yet distinctly discrete, as the duo developed careers in radio astronomy and astrophysics that began with pulsars, then included FRBs and now envelop gravitational waves. The couple have also advanced science education and grown astronomical research and teaching at their home base, West Virginia University (WVU) in the US. There, McLaughlin is Eberly Family distinguished professor of physics and astronomy, and chair of the Department of Physics and Astronomy, while Lorimer currently serves as associate dean for research in WVU’s Eberly College of Arts and Sciences.
The Shaw Prize
The 2023 Shaw Prize in Astronomy, awarded jointly to Duncan Lorimer and Maura McLaughlin, and to their colleague Matthew Bailes, is part of the legacy of Sir Run Run Shaw (1907–2014), a successful Hong Kong-based film and television mogul. Known for his philanthropy, he gave away billions in Hong Kong dollars to support schools and universities, hospitals and charities in Hong Kong, China and elsewhere.
In 2002 he established the Shaw Prize to recognize “those persons who have achieved distinguished contributions in academic and scientific research or applications or have conferred the greatest benefit to mankind”. A gold medal and a certificate for each Shaw laureate, and a monetary award of $1.2m shared among the laureates, is given yearly in astronomy, life science and medicine, and mathematical sciences. Previous winners of the Shaw Prize in Astronomy include Ronald Drever, Kip Thorne and Rainer Weiss, for the first observation of gravitational waves with LIGO. They are among the 16 of the 106 Shaw laureates since 2004 who have also been awarded Nobel prizes.
Accidental cosmic probe
Radio astronomy, which led to much of McLaughlin and Lorimer’s work, was not initially a formal area of research. Instead, it began rather serendipitously in 1928, when Bell Labs radio engineer Karl Jansky was trying to find the possible sources of static at 20.5 MHz that were disrupting the new transatlantic radio telephone service. Among the types of static that he detected was a constant “hiss” from an unknown source that he finally tracked down to the centre of the Milky Way galaxy, using a steerable antenna 30 m in length. His 1933 paper “Electrical disturbances apparently of extraterrestrial origin” received considerable media attention but little notice from the astronomy establishment of the time (see “Radio astronomy: from amateur roots by worldwide groups” by Emma Chapman).
Radio astronomy truly flourished after the Second World War, with new purpose-built facilities. An early example from 1957 was the steerable 76 m dish antenna built by Bernard Lovell and colleagues at Jodrell Bank in the UK – where McLaughlin and Lorimer would later work. Other researchers who led the way include the Nobel-prize-winning astronomer Sir Martin Ryle, who pioneered radio interferometry and developed aperture synthesis; as well as Australian electrical engineer Bernard Mills, who designed and built radio interferometers.
Extraterrestrial radio signals soon yielded important science. In 1951 researchers detected a predicted emission from neutral hydrogen at 1.4 GHz – a fingerprint of this fundamental atom. In 1964 Arno Penzias and Robert Wilson (also based at Bell Labs) inadvertently found a 4.2 GHz signal across the whole sky, while testing orbiting telecom satellites – thereby discovering the cosmic background radiation. And in 1968 another spectacular discovery shaped McLaughlin and Lorimer’s careers, when University of Cambridge graduate student Jocelyn Bell Burnell and her PhD supervisor Antony Hewish announced the observation of an unusual radio signal from space – a pulse that arrived every 1.3 seconds. That signal was the first to come from what were soon called “pulsars”. Hewish would go on to share the 1974 Nobel Prize for Physics for the discovery – while Bell Burnell was infamously left out, supposedly due to her then student status.
As more pulsars were found with varied periods and in different directions of the sky, it became clear that the signals were not being sent by an alien civilization as some researchers had speculated – after all, the chances of an extraterrestrial civilization sending many signals of varying periods, or different civilizations sending out different periodic signals, was slim. One clue was that the pulses were short and coherent, so they had to come from sources smaller than the distance light could travel during the pulse’s lifetime – for instance, the source of a 5 ms pulse could be at a maximum of 1500 km.
As it happened, the signals were our first look at neutron stars – small, extremely dense and rapidly rotating remnants of massive stars after they have gone supernova and had their protons and electrons squeezed into neutrons by gravity’s implacable power. As the star rotates, its strong off-axis magnetic field produces beams of electromagnetic radiation from the magnetic poles. These beams create regular pulses as they sweep past a detector on a direct line of sight. Pulsars are mostly studied at radio frequencies, but they also radiate at other, higher frequencies.
Pulsars to fast bursts
Lorimer and McLaughlin began their careers by studying these exotic stellar objects, but each of them had already been captivated by astronomy and astrophysics as teenagers. Lorimer was born in Darlington, UK. After studying astrophysics as an undergraduate at the University of Wales in Cardiff, he moved to the University of Manchester in 1994, where his PhD research focused on analysing classes of radio pulsars with different periods.
McLaughlin was born in Philadelphia, Pennsylvania, and first studied pulsars as an undergraduate student at Penn State. Her PhD dissertation at Cornell University in 2001 covered pulsars that variously emitted radio waves, X-rays or gamma rays. By 1995 Lorimer was working as a researcher at the Max Planck Institute for Radio Astronomy in Bonn, Germany, whereas McLaughlin joined the Jodrell Bank Observatory in 2003. He met McLaughlin in 1998 while working at the Arecibo Observatory in Puerto Rico. McLaughlin and Lorimer moved to the UK in 2001 to work at the Jodrell Bank observatory.
It was an interesting and exciting time in the pulsar research community, with new pulsars found by computerized Fourier transform analysis that detected the telltale periodicities in vast amounts of observational data. But radio astronomers also sometimes saw transient signals, and McLaughlin had written computer code designed to find single bright pulses. This led to the 2006 discovery of a new class of pulsars dubbed rotating radio transients (RRATS, an acronym recalling a pet rat McLaughlin once had). These stars could be detected only through their sporadic millisecond-long bursts, unlike most pulsars, which were found through their periodic emissions. The discovery in turn initiated further searches for transient pulses (Nature 439 817).
The following year, Lorimer and McLaughlin, now a married couple, joined WVU’s department of physics and astronomy as assistant professors. To uncover more distant and bright pulsars, Lorimer gave his graduate student Ash Narkevic the task of looking through archival observational data that the Parkes radio telescope in Australia had taken of the Large and Small Magellanic Clouds – two small galaxies that are satellites to our very own Milky Way, roughly 200,000 light-years away from Earth – of which the Large was already known to host 14 pulsars.
Narkevic examined the data and found a single strong burst – nearly 100 times stronger than the background – at 1.4 GHz with a 5 msec duration. But the burst seemed to come from the Small Magellanic Cloud, where there were five known pulsars at that time. Even more surprising was the fact that this extremely bright burst did not arrive all the same time. Known as pulse or frequency dispersion, this occurs when radio waves travelling through interstellar space interact with free electrons, dispersing the waves, as higher-frequency waves travel through the free-electron plasma quicker than lower-frequency ones, and arrive earlier at our telescopes.
This dispersion depends on the total number of electrons (or the column density) along the path. The further away the source of the burst, the more likely it is that the waves will encounter even more electrons on their path to Earth, and so the lag between the high- and low-frequency waves is greater. The pulse Narkevic spotted was so distorted by the time it reached Earth that it suggested the source was almost three billion light-years away – well beyond our local galactic neighbourhood. This also meant that the source must be significantly smaller than the Sun, and more on par with the proposed size of pulsars, while also somehow being 1012 times more luminous than a typical pulsar.
1 The first burst
(Top) Duncan Lorimer (left) and Ash Narkevic in 2008 with the paper they published in Science about their observation of a fast radio burst (bottom).
The report of this seemingly new phenomenon – a single extremely energetic event at an enormous cosmological distance – was published in Science later that year, after being initially rejected (Science 318 777). This first detected fast radio burst came to be known as the “Lorimer burst” (figure 1). After several years and significant further work by Lorimer, McLaughlin, Bailes and others, they found first four and then tens of similar bursts. This launched a new class of cosmological phenomena that now includes more than 1000 FRBs, which have fulfilled the prediction in 2007 that they would serve as cosmological probes.
Thanks to FRBs having been found in different galaxies beyond our own across the sky, they serve as a probe of the intergalactic medium, allowing astrophysicists to measure the density of the material that lies between Earth and the host galaxy (Nature 581 391). By measuring the distance to the source of the FRB, and then looking at the dispersion as a function of wavelength of the pulses, astronomers can determine the density of the matter the pulse passed through, thereby yielding a value for the baryonic density of our universe. This is otherwise extremely difficult to measure, thanks to how diffused this matter is in our observable universe. FRBs have also provided an independent measurement for the Hubble constant, the exact value of which has lately come under new scrutiny (MNRAS 511 662).
Detecting a gravitational-wave background
While Lorimer is still working on pulsars and FRBs, McLaughlin has now moved into another area of pulsar astronomy. That’s because for almost two decades, she has been a researcher in and co-director of the North American Nanohertz Observatory for Gravitational Waves (NANOGrav) Physics Frontier Center, which uses pulsars to detect low-frequency gravitational waves with periods of years to decades. One of its facilities is the steerable 100 m Green Bank Telescope about 150 km south of WVU.
“We are observing an array of pulsars distributed across the sky,” says McLaughlin. “These are 70 millisecond pulsars, so very rapidly rotating. We search for very small deviations in the arrival times of the pulsars that we can’t explain with a timing model that accounts for all the known astrophysical delays.” General relativity predicts that certain deviations in the timing would depend on the relative orientation of pairs of pulsars, so seeing this special angular correlation in the timing would be a clear sign of gravitational waves.
2 Gravitational-wave spectrum
(a) The NANOGrav 15-year data set contains timing observations from 68 pulsars using the Arecibo Observatory, the Green Bank Telescope and the Very Large Array. The map shows pulsar locations in equatorial co-ordinates. (b) The background comes from correlating changes in pulsar arrival times between all possible pairs of the 67 pulsars (2211 distinct pairs in total), and is based on three or more years of timing data. The black line is the expected correlation predicted by general relativity. These calculations assume the gravitational-wave background is from inspiralling supermassive black-hole binaries.
In June 2023 the NANOGrav collaboration published an analysis of 15 years of its data (figure 2), looking at 68 pulsars with millisecond periods, which showed this signature for the first time (ApJL 951 L8). McLaughlin says that it represents not just one source of gravitational waves, but a background arising from all gravitational events such as merging supermassive black holes at the hearts of galaxies. This background may contain information about how galaxies interact and perhaps also the early universe. Five years from now, she predicts, NANOGrav will be detecting individual supermassive black-hole binaries and will tag their locations in specific galaxies, to form a black hole atlas.
Star-crossed astronomers
The connections between McLaughlin and Lorimer that played a role in their academic achievements began rather fittingly with an interaction in 1999, at the Arecibo radio telescope in Puerto Rico (now sadly decommissioned). Lorimer was based there at the time, while McLaughlin was a visiting graduate student, and their contact, though not in person, was definitely not cordial. Lorimer sent what he calls a “little snippy e-mail” to McLaughlin about her use of the computer that blocked his own access, which she also recalls as “pretty grumpy”.

But things improved after they later met in person, and they joined the Jodrell Bank Observatory in the UK. The pair married in 2003 and now have three sons. Over the years, they moved together to the US, set up their own astronomy group at WVU by 2006, and proceeded to work together and alongside each other, publishing many research papers, both joint and separate.
Given all these successes, how do the two researchers balance science and family, especially when they first arrived at WVU with a five-month-old baby to join a department with just one astronomer and no graduate astronomy programme? McLaughlin says it was “Really hard work. Lots of grant writing, developing courses,” but adds that it was also “really fun because we were both building a programme and building a family and moving to a new place”.
Life got even busier in 2007, when another child and the FRB discovery both arrived. The couple says that it was all doable because they fully understood the need to shift scientific or family responsibilities to each other as necessary. According to McLaughlin, this includes equal parenting from her husband, for which she feels “very lucky”. As Lorimer puts it, “We get each other’s mindset.”
However, the fact that they are married may have coloured perceptions of their work and status. “When we first started here at WVU,” Lorimer explains, “a lot of people assumed we were sharing a single position. But the university’s been great. It’s always made it clear from the get-go that we’re obviously on different career trajectories.” And they agree that as they’ve progressed in their individual careers and are known for different things, they’re now unmistakably seen as two distinct scientists.
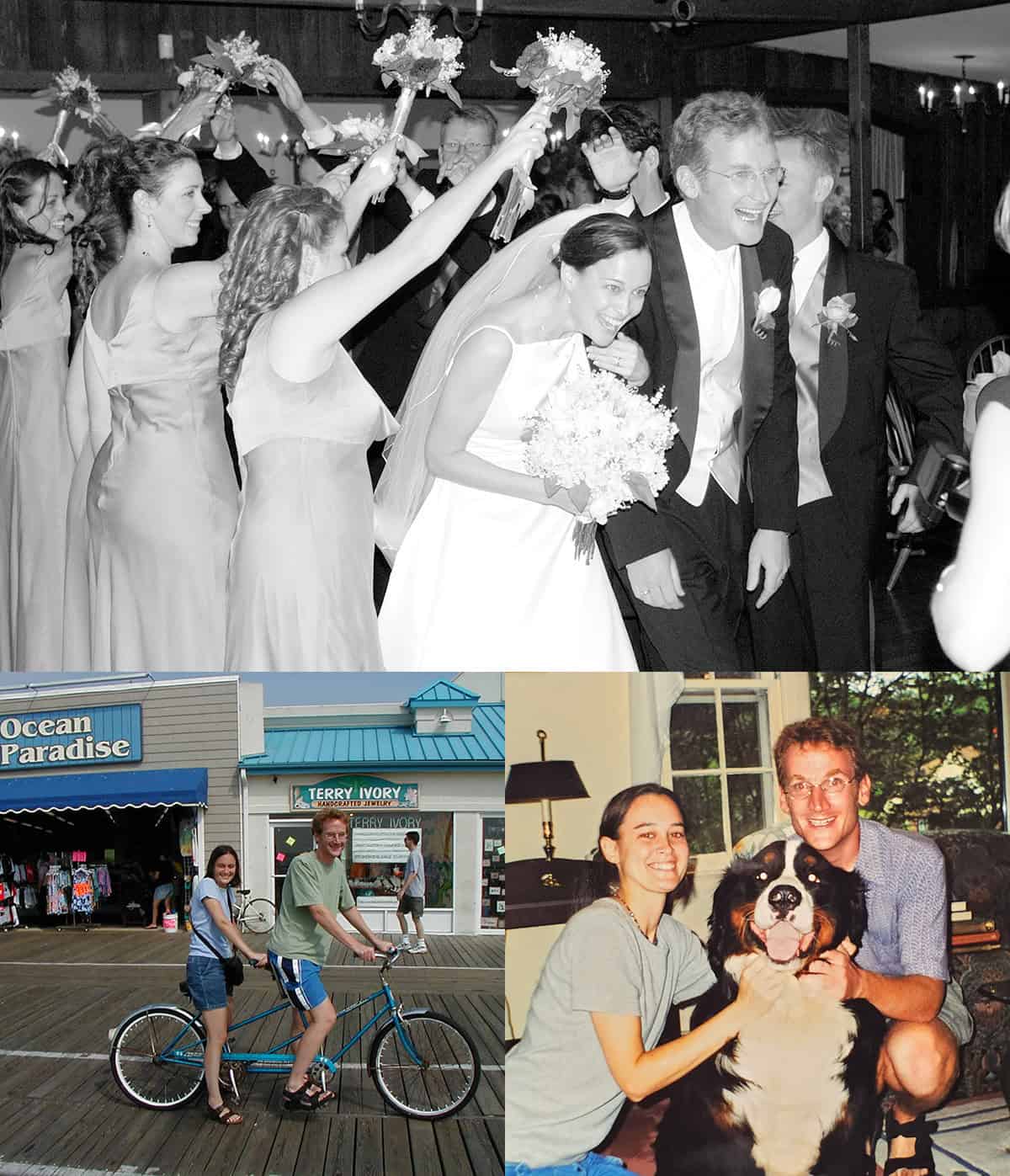
Beyond the Shaw Prize
The Shaw Prize came as a total surprise to the couple. The pair both received e-mails simultaneously one evening, but Lorimer spotted his first. “We almost missed it as it was just about time to go to bed and the announcement was being made in Hong Kong a few hours after that,” says Lorimer. McLaughlin recalls her husband screaming and excitedly running up the stairs to give her the news. “He doesn’t scream much to begin with, maybe only when the dogs do something bad, and I’m wondering ‘Why is he screaming late on a Sunday night?’ He told me to pull up the e-mail and I thought it was a prank. I read it again and realized it was real. That was quite a Sunday night.” Amusingly, the e-mail for their co-winner Matthew Bailes initially went into his spam folder. The trio would later describe their work in a Shaw Prize Lecture in Hong Kong in November 2023.
So what comes next for the stellar pair? Further research into the different types of FRBs that are still being found, using new telescopes and detection schemes. One new project, an extension of Lorimer’s earlier work in pulsar populations, is to locate FRBs in specific galaxies and among groups of both younger and older stars using the Green Bank telescope in West Virginia, along with others, to help uncover what causes them. FRBs may come from neutron stars with especially huge magnetic fields – dubbed magnetars – but this remains to be seen.
Data from Green Bank is also used in the Pulsar Science Collaboratory, co-founded by McLaughlin and Lorimer (see box below). Meanwhile, the NANOGrav pulsar observation of the gravitational wave background, where McLaughlin continues her long-time involvement, has been hailed by the LIGO Collaboration for opening up the spectrum in the exciting new era of gravitational-wave astronomy and cosmology.
The Pulsar Science Collaboratory
The Pulsar Science Collaboratory (PSC) was founded in 2007 by Maura McLaughlin, Duncan Lorimer and Sue Ann Heatherly at the Green Bank Observatory; with support from the US National Science Foundation. It is an educational project in which, to date, more than 2000 high-school students have been involved in the search for new pulsars.
Students are trained via a six-week online course and then must pass a certification test to use an online interface to access terabytes of pulsar data from the Green Bank Observatory. They are also invited to a summer workshop at the observatory. McLaughlin and Lorimer proudly note the seven new pulsars that high-school students have so far discovered. Many of these students have continued as college undergraduates or even graduate students working on pulsar and fast-radio-burst science.
At the end of the Shaw Prize Lecture, Lorimer pointed out that there is “still much left to explore”. In an interview for the press, McLaughlin said “We’ve really just started.” Both statements seem fair predictions for anything each one does in their areas of interest in the future – surely with hard work but also with the continuing sense that it’s “really fun”.