
Particle therapy is usually delivered using a large and costly gantry to change the angle of incidence of the therapeutic ion beam relative to the patient. If the patient were rotated instead, a simpler fixed-beam configuration could provide 360° access for the particle beam. During patient rotation, however, the changing direction of the gravitational force will deform and displace the tumour and surrounding organs in an unpredictable way. To ensure precise dose delivery to the tumour, such anatomical changes must be detected and compensated for during irradiation.
“Image guidance is absolutely necessary for particle therapy with patient rotation,” explains Kilian Dietrich from Heidelberg University Hospital and the German Cancer Research Center (DKFZ). “To exploit the main benefit of particle therapy – high dose escalation at the tumour with minimal dose to surrounding healthy tissue – prior knowledge of the tissue composition in the irradiation path is required.”
In conventional photon-based radiotherapy, MRI can be implemented in so-called MR-linacs, which offer the possibility to visualize changes in anatomy or patient position with high soft-tissue contrast. However, combining MRI with particle therapy including patient rotation remains a significant challenge.
Particle beams of protons, carbon ions or helium ions are extremely sensitive to non-homogeneous materials in the irradiation path, placing constraints on the MRI magnet and components. To address these limitations, Dietrich and colleagues are developing a radiation-transparent body coil to enable MR-guided particle therapy in combination with patient rotation, describing their work in Medical Physics.
Radiation transparency
One key obstacle when integrating MRI with particle therapy is the design of the radiofrequency (RF) coils used to flip the magnetization of the tissue and receive the generated MR signals. Conventional imaging coils contain highly attenuating electronic components that, if located in the beam path, will cause ion attenuation and scattering that alter the delivered dose distribution and reduce treatment efficacy.
To prevent such adverse effects, the team designed an RF coil with minimal ion attenuation, based on a cylindrical 16-rung birdcage configuration. This specific birdcage coil only has capacitors on the end rings, thereby avoiding attenuation and scattering in a large window in between. And since the birdcage functions both as a transmit and a receive coil, no additional RF coils are required. The design also allows easy integration into a capsule that enables rotation of the patient and the coil together, providing 360° access for a fixed ion beam source.
The researchers built the RF coil from a 35 µm-thick copper conductor embedded between layers of flexible polyimide and adhesive. The coil has an inner diameter of 53 cm and an axial length of 52 cm – providing a large enough field-of-view for full-body cross section imaging.
Measuring the Bragg peak shift caused by the entire RF coil confirmed its total water equivalent thickness (WET, a measure of ion attenuation) as 420 µm. This includes the polyimide and adhesive layers, which are homogeneous and can be compensated for with higher particle beam energy. The WET of the copper layer alone, which is inhomogeneous and cannot simply be compensated for, was approximately 210 µm. This is well within the clinical precision required for dose planning, which lies in the order of millimetres. As such, the team classifies the RF coil as radiation transparent.
Effective imaging
To characterize the imaging quality of their RF coil, the researchers imaged a homogeneous tissue-simulating phantom using a 1.5 T MR system. For the three central planes in the phantom, the transmit RF field distributions were homogeneous and resembled those of simulations and the MR system’s internal body coil. The measured transmit power efficiencies (between 0.17 and 0.26 µT/√W) were lower than the simulated values, but exceeded those of the internal body coil.
To examine the impact of coil rotation, they determined the mean transmit power efficiency in a central subvolume of the phantom for a full capsule rotation. Compared with the simulations, the measurements showed a slight dependence on rotation angle, with optimal transmit power efficiency at rotation angles close to 0° and 180°.
The RF coil also exhibited uniform signal acquisition in the three central phantom planes, with similar receive sensitivity profiles as observed in the simulations, both with the phantom in the horizontal position and when rotated by 30°. For a full rotation of the capsule, the measured receive sensitivity varied between 62% and 125%, decreasing at rotation angles between 15° and 120° and at 205°.
The signal-to-noise ratio (SNR) of the RF body coil showed a slight dependence on the rotation angle, ranging between 103 and 150. Overall, an increase of 10%–43% over the SNR of the internal body coil was achieved, indicating reasonable imaging quality for thoracic, abdominal and pelvic MRI.
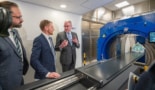
OncoRay launches world’s first whole-body MRI-guided proton therapy system
To estimate the effect of realistic patient loading in the RF coil, the team also simulated a heterogeneous human voxel model, observing high transmit power efficiency and receive sensitivity for all rotation angles. The next step will be to perform in vivo measurements.
“The RF coil has not been tested in vivo yet since further tests are necessary before the whole setup can be tested,” Dietrich tells Physics World. “This includes patient acceptance for the rotation system as well as the time required to rescue the patient in times of emergency.”