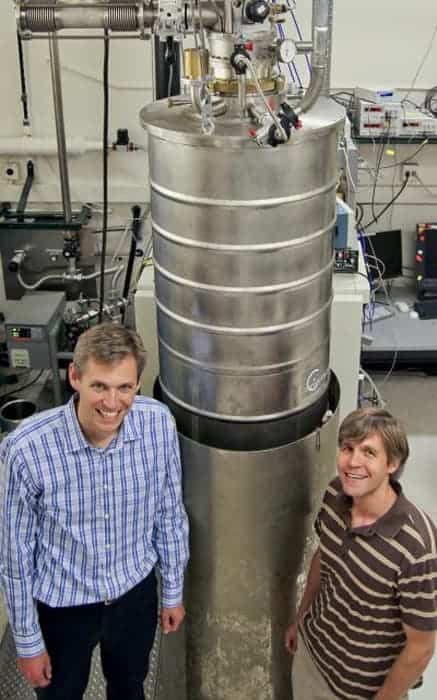
Researchers in the US have entangled the motion of a tiny mechanical drum with a microwave field. This is the first time that a macroscopic oscillator has been entangled and the work extends the observation of quantum behaviour to larger objects than before. The researchers hope to apply their results in the creation of a quantum computing circuit.
Cool beats
In early 2010, researchers at the University of California in the US were the first to have observed true quantum behaviour in a macroscopic object that was cooled down to its quantum ground state. Then, in 2011, Konrad Lehnert and colleagues at JILA – a joint institute of the University of Colorado at Boulder and the National Institute of Standards and Technology (NIST) – followed on from that work and cooled a similar microdrum down to the ground state with a technique known as “sideband cooling”, which uses microwaves instead of laser light. They cooled the drum to below 400 μK, steadily lowering its energy to just one-third of one quantum.
Then in March this year, Lehnert’s group was the first to store and retrieve quantum information from their oscillator by connecting it to a microwave circuit. In that experiment, the researchers transferred the states of a microwave field into their mechanical oscillator and then converted the state of the oscillator back into a microwave field. Now, Lehnert, along with his postdoctoral researcher Tauno Palomaki and colleagues have extended this by creating an entangled state between the oscillator and a microwave field by exciting the circuit above its resonance frequency. “During the entanglement process a microwave pulse emerges from the circuit but unlike the previous work, it’s not a state that was stored in the mechanical oscillator. That pulse is generated spontaneously by the entanglement process,” explains Lehnert. The microwave field that emerges from the circuit as a result of their experiment is known as the “pulse”, while the microwave field that they impose on the electromechanical circuit is known as the “pump”.
Perfect tuning
Lehnert tells physicsworld.com that if the pump is tuned above the circuit’s resonance frequency, a spontaneous process creates a pulse and places the mechanical oscillator in a state that is correlated with that pulse. “If the mechanical oscillator is near its ground state when that pump is applied, the oscillator and pulse won’t just be correlated, they should be entangled,” explains Lehnert.
In the experiment, the first pump is tuned below the circuit’s resonance, transferring the thermal state of the oscillator into a pulse that removes the thermal energy from the drum, cooling it to near its ground state. The second pump tone is tuned above the circuit’s resonance and this creates a pulse that is entangled with the oscillator. The third and final pump is, once more, tuned below resonance and it converts the state of the oscillator into a third pulse. During this process, a train of three pulses emerges from the circuit. The first contains the thermal state of the oscillator but it is the second and third pulses that are of interest to the researchers. “We show that the second and third pulses are entangled and because we know that the third pulse contains the state of the oscillator immediately after the entanglement was generated, the oscillator must have been entangled with the second pulse,” says Lehnert.
Subtly entangled
Lehnert is clear that that there is, undoubtedly, some subtlety when it comes to a demonstration of entanglement itself. “Evidence of entanglement comes from asking how well we can anticipate the measurement of the third pulse, based on our measurement of the second. That’s a good operational notion of correlation,” he says. If the pulses are indeed entangled, a measurement of the second pulse should allow the team to anticipate the outcome of the measurement of the third, with an uncertainty that is smaller than the fluctuations associated with the quantum vacuum fluctuations (a temporary change in the amount of energy in a point in space that comes about due to Heisenberg’s uncertainty principle).
Lehnert explains that the subtlety comes into play because the state of a pulse is specified by two numbers – the real and imaginary components. These two numbers obey the uncertainty principle – either can be measured with arbitrary precision but if both are measured simultaneously, the measurement itself introduces noise that is at least as large as the vacuum fluctuations. But for a demonstration of entanglement, the team must measure the correlations both in the real part and in the imaginary parts and it did so by measuring both simultaneously. “As such we must carefully characterize the noise added by the measurement itself. Having done so, we can show that the second and third pulses are entangled,” says Lehnert. According to him, the team has a high degree of confidence that the oscillator and the microwave pulse are entangled each time the experiment is carried out.
The US researchers are now looking to combine their circuit with superconducting qubits to store and retrieve a qubit state from the oscillator, as their work demonstrates an essential requirement for using compact and low-loss micromechanical oscillators for quantum processor. “In addition, we’d also like to use devices of this type to build quantum-enhanced force sensors. That is, we’d like to show that we can use entanglement to circumvent the quantum noise that would otherwise limit the sensitivity of a force measurement,” says Lehnert.
The research is published in Science Express.