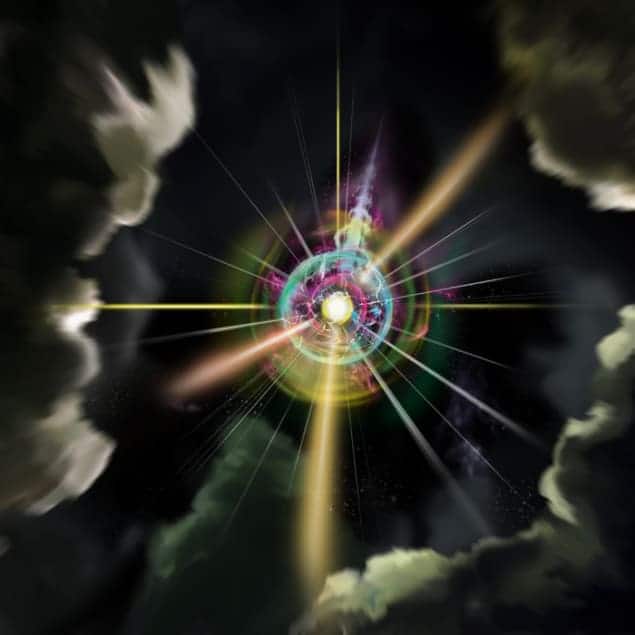
An analogue of a long-sought-after particle comprising an isolated magnetic pole has been observed by physicists in the US and Finland. “Magnetic monopoles” were predicted by Paul Dirac in 1931 but have never been seen in nature. This latest work does not prove whether or not the unusual particles exist, rather it shows that a physical system described by the underlying mathematics can be created in the lab. The research could also help physicists to gain a better understanding of exotic materials such as superconductors, and even create materials with new and useful properties.
Magnetic poles are always seen in pairs, no matter how small the magnet. An ordinary bar magnet consists of both a north and a south pole; if the magnet is cut in two, then each of the resulting halves will also be bipolar. In fact, no matter how many times the magnet is divided, the north and south poles remain coupled – even as far down as individual atoms, which themselves act like tiny magnets. This is reflected in Maxell’s equations, which say that isolated positive and negative electric charges exist but isolated magnetic charges do not.
This changed when quantum mechanics was formulated in the early 20th century. Dirac showed that naturally occurring magnetic monopoles would require electric charge to come in discrete units. This discreteness is seen in nature but is not fully understood, and therefore the search for magnetic monopoles is an active field of research.
Searching high and low
So far, physicists have tried to create monopoles inside particle accelerators, but the monopole mass is generally considered too high to allow a sighting, even at CERN’s Large Hadron Collider. Another option was to search pristine environments, such as the Moon or Antarctic ice, for signs of the monopoles that grand unified theories predict should have been created as the universe cooled and its initial symmetry was broken. Here too, however, researchers have come up empty-handed.
The approach of David Hall and colleagues at Amherst College in Massachusetts and collaborators at Aalto University in Finland is to produce an analogue of what is known as a “Dirac monopole”, the generalized quantum-mechanical form of a magnetic monopole put forward by Dirac. Prior to 1931, nobody had been able to combine classical electromagnetism and quantum mechanics to allow the existence of magnetic monopoles, but Dirac was able to do this by considering what happens when a monopole interacts with an electron. He found that when a monopole passes through an electron cloud – the distribution in space of a single electron as described by quantum mechanics – it leaves a vortex in its wake. This is a line of zero electron density around which the density spirals: “Like water swirling as it goes down the drain,” says Hall.
Hall’s group has reproduced that vortex in a Bose–Einstein condensate of ultracold rubidium atoms. The condensate is a single matter wave and stands in for the electron cloud in Dirac’s formulation. To reproduce the monopole, the researchers applied a real, external magnetic field to the condensate to orient the constituent atoms in such a way that they create a “synthetic” magnetic field inside the condensate. There is a “one-to-one correspondence” between that synthetic field and the field that would be produced by a magnetic monopole, Hall explains. “You could draw exactly the same field lines in the synthetic field and the locus of the monopole is where those field lines spring from,” he says.
Polar vortex
To show that they really had produced a Dirac monopole, the researchers shone a laser beam through the condensate. The beam created a “shadowgraph”, in which the shadow cast by the atoms in the sample was pierced by a narrow strip of light. That strip, they concluded, was the vortex created by an isolated north pole (it being north rather than south simply for technical reasons). “What we see is remarkable,” says Hall, “because normally a vortex created inside a Bose–Einstein condensate goes from one side of the condensate to another. But here it ends in the bulk. That is the hallmark of the monopole.”
Will it help particle physicists find real monopoles? Probably not, but it should encourage them to keep looking
Peter Holdsworth, Ecole Normale Supérieure, Lyon
Peter Holdsworth, a condensed-matter physicist at the Ecole Normale Supérieure in Lyon, praises the work as “an exquisite application of nanotechnology, cold atoms, high-powered computing and clever theory”. He points out that the US–Finnish team has not proved the existence of magnetic monopoles, but he thinks the researchers have provided experimental confirmation of Dirac’s mathematics. “It is an important result and could lead to many other analogous results,” he says. “Will it help particle physicists to find real monopoles? Probably not, but it should encourage them to keep looking.”
Insight into natural monopoles
Hall is quick to acknowledge the limits of his group’s work. “Our monopoles wouldn’t be registered by a compass,” he says. “We haven’t been able to reproduce properties such as the mass of the particle in our experiment, but we have created an analogue of the magnetic part. That might provide some insight into natural monopoles.”
Hall argues that his group has come closer to imitating would-be natural magnetic monopoles than three other groups that reported results on materials known as spin ices in 2009. In that earlier work, the tetrahedra-shaped collections of ions that make up spin ices were observed under certain conditions to acquire net spin, so resembling either isolated north or south poles. Hall describes these experiments as “beautiful” but maintains that the connection to Dirac monopoles was fairly weak as the phenomenon in question was purely classical, as opposed to quantum.
Real magnetic charge
Holdsworth, who works on spin-ice physics, takes a different view. He argues that the spin-ice systems provide a strong parallel with natural monopoles because, he says, “the charge there really is magnetic and they really do produce magnetic fields”.
As far as applications are concerned, Hall believes that his group’s work could help physicists to perform quantum simulations of matter. This fast-growing field, he explains, aims to understand existing materials and ultimately create new ones, perhaps even room-temperature superconductors. Quantum simulations use ultracold atoms to represent electrons – the atoms jumping around an optical lattice just as electrons move between ions. “You need a synthetic electric or magnetic field to talk to those atoms as if they were electrons,” says Hall. “The synthetic fields that we are creating could be used for this.”
The research is reported in Nature.
- The 2009 spin-ice experiments are described in two news articles: “‘Magnetic charge’ measured in spin ice” and “Magnetic monopoles spotted in spin ices“