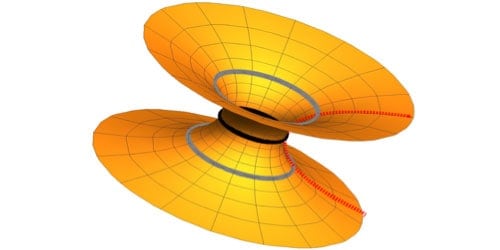
Earlier this year, researchers working on the Advanced Laser Interferometer Gravitational-wave Observatory (aLIGO) made the first ever detection of gravitational waves. The waves are believed to have been created by the merger of two binary black holes, in an event dubbed GW150914. Now, however, new theoretical work done by an international team of researchers suggests that other hypothetical exotic stellar objects – such as wormholes or “gravastars” – could produce a very similar gravitational-wave signal. While it is theoretically possible to differentiate between the different sources, it is impossible to tell whether GW150914 had a more exotic origin than merging black holes because the signal was not strong enough to be resolved.
The researchers point out that, in the future, the detection of stronger gravitational-wave signals could reveal more information about their sources – especially once the sensitivity of aLIGO is increased to its ultimate design level. In addition, future space-based detectors, such as the European Space Agency’s Evolved Laser Interferometer Space Antenna (eLISA), could reveal tiny discrepancies between detected and predicted signals, if they exist.
Ringing frequencies
Einstein’s general theory of relativity provides a very clear theoretical framework for the type of gravitational-wave signal that would be produced during the collision and subsequent merger of massive, compact bodies, such as black holes. Gravitational waves are produced constantly before, during and just after a merger. The waves’ frequencies will vary, telling us when the black holes’ orbit begins to reduce and they begin their slow inward collapse, or “inspiral”. The smaller the initial distance between the two, the more radiation is emitted as the black holes plunge into one another. This produces a characteristic “chirp” waveform, wherein the frequency and the amplitude of the waves increase until they peak at the merger.
But such a cataclysmic merger initially gives birth to a highly distorted black hole, which rids itself of its deformity almost instantly, by ringing like a bell and producing further gravitational radiation. The system quickly loses energy and the strength of the waves decays exponentially to form a “ringdown” signal, all of which was picked up by aLIGO for GW150914.
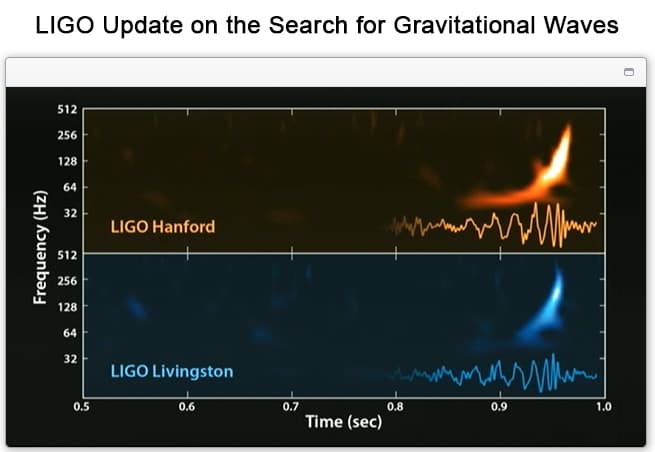
The chirp and the ringdown signal are of immense interest as these carry crucial information about the mass and spin of both the initial black holes, and of the newly formed one. “This ringdown phase is very important: just as a Stradivarius violin vibrates in a characteristic way, so too do black holes. Thus, by studying carefully how it rings, you hope to know the black hole itself,” says physicist Vitor Cardoso from the University of Lisbon, Portugal.
These vibrational modes of a nascent black hole – known as quasinormal modes – must be detected within the signal, to be absolutely certain that the gravitational waves have arisen from coalescing black holes. Our current understanding suggests that these vibrational modes are inherently linked to a black hole’s key feature – its event horizon, or the boundary past which nothing, not even light, can escape from its gravitational pull.
Light rings
But new simulations and analysis – carried out by Cardoso together with team members Paolo Pani and Edgardo Franzin – have shown that a virtually indistinguishable ringdown signal can be produced by a “black-hole mimicker”, thereby potentially allowing us to detect these exotic objects. These mimickers are hypothetical objects that could be as compact as black holes but do not have an event horizon. They could be gravastars – celestial objects whose interior is made of dark energy – or wormholes – a tunnel through space–time connecting two distant regions of the universe.
These exotic objects possess “light rings”, which are yet another artefact of general relativity – a circular photon orbit which is predicted to exist around very compact objects. “A light ring is very different from an event horizon, because signals can escape from regions within the light ring – although they would be highly red-shifted – whereas nothing can escape from the event horizon,” explains Cardoso. All compact objects would in theory possess a light ring. Indeed back holes have one that is associated with the border of their silhouette. These are the so-called “black-hole shadows” that lie just outside of their event horizon. On the other hand, neutron stars, while very compact, are not compact enough to develop a light ring.
Cardoso and colleagues looked into objects with only light rings and found that “if an object is compact enough to possess a light ring, then the ringdown would be almost identical to that of a black hole. The more compact the object, the more similar the ringdown”. Indeed, the team’s simulations showed that the ringdown signal is mostly associated with the light ring. It is the light ring itself that is vibrating, not the event horizon.
Mimicking wormholes
The team’s simulations calculated this explicitly for a wormhole, but Pani told physicsworld.com that the “same result is valid for gravastars and, as we claim, for all ultracompact black-hole mimickers”. But the researchers’ analysis also showed that these mimickers eventually leave an imprint in the gravitational-wave signal in the form of “echoes”, which are reflections of the waves from the surface of these objects. “These echoes may take a long time to reach our detectors, so it is important to scrutinize the data even long after the main pulse has arrived,” says Cardoso. More precisely, the mimicker signal will ultimately deviate from that predicted for a black hole, but only at late times.
LIGO scientist Amber Stuver, who is based at the LIGO Livingston Observatory in Louisiana, US, is “thrilled” by the possibility that aLIGO may have detected an exotic object, but she confirms that “there is nothing in our observations that is inconsistent with this being a normal stellar mass black-hole system possessing an event horizon. Until we have evidence otherwise, we can’t claim that this was anything but a stellar mass black hole binary merger.” She tells physicsworld.com that “advanced detectors such as aLIGO, aVirgo, and KAGRA will need to increase their sensitivity” to pick up such signals. She also points out that the GW150914 event “was detected with aLIGO at about 30% of its design sensitivity. The potential is real that, if these exotic horizonless objects are out there mimicking black holes, we may very well find them in the near future”.
B S Sathyaprakash from Cardiff University in the UK, who is also a part of the LIGO team, agrees with the theorists’ work, saying that “Our signal is consistent with both the formation of a black hole and a horizonless object – we just can’t tell.” He further explains that, although Einstein’s equations predict how slightly deformed black holes vibrate, our understanding is incomplete when their deformation is large. “That’s why we need a signal in which the post-merger oscillations of the merged object are large, and this can happen if we detect even more massive objects than GW150914, or if GW150914 is at least two to four times closer.” Then, it would be possible to distinguish the signals, he says.
Cardoso acknowledges that “black-hole mimickers are very exotic objects and by far black holes remain the most natural hypothesis”. But he adds: “It is important to understand whether these exotic objects can be formed (for example in a stellar collapse) and if they are stable. Most importantly, we only focused on the ringdown part, but it is equally relevant to explain the entire gravitational-wave signal, including the inspiral and the merger phases. This would require performing numerical simulations with supercomputers to understand whether this picture is viable or not. We are currently working on this.”
The research is published in Physical Review Letters.