
A five-qubit trapped-ion quantum computer, which is programmable and reconfigurable, has been demonstrated by researchers from the Joint Quantum Institute in the US. The team’s computing architecture is such that the researchers can programme multiple algorithms into their trapped-ion processor, which is a first. Although the computer is relatively small at five qubits and the algorithms they process fairly simple, the researchers say that there are a variety of ways to scale up this architecture to build a functional quantum computer in the future.
The hallmark of a quantum computer will be its ability to solve certain computational problems – such as factoring large numbers or simulating complex chemical reactions as well as the interactions between large numbers of fundamental particles – exponentially faster and more efficiently than is possible with current classical computing. There are a variety of quantum methods and technologies – including superconducting qubits and trapped ions or quantum annealers and adiabatic quantum computing – that various groups around the world are adapting in the race towards building the first true quantum computer.
Five-ion trap
Chris Monroe’s group at the Joint Quantum Institute and the Joint Center for Quantum Information and Computer Science, at the University of Maryland in the US, uses trapped ions as qubits. In this technique, information is stored in the atomic-ions’ states. Electromagnetically confining a number of such ions, or “trapping” them, the particles can then be entangled by applying appropriate laser beams. The finely tuned laser light manipulates each ion in a specific way, depending upon its state. “In this way, the collective motion of the chain of ions behaves as a data bus that allows qubits to talk to each other,” say Monroe.
These operations are the quantum logic gates in the system, and with ions, the researchers are able to execute gates between any pair of ions in the chain. Monroe further explains that the effective wiring of the quantum computer in this case is enforced from the outside – this is a unique feature of ion qubits, he adds, because “they are not hard-wired and hence their connections can be reconfigured and programmed from the outside”.
While small ion-trap quantum computers have previously been built, each was a single-purpose device, capable of running a particular algorithm or generating a fixed entangled state. Now though, Monroe, together with Shantanu Debnath and colleagues, has demonstrated that the device can be programmed with multiple algorithms.
The team’s processor is made up of five ytterbium ions confined in a linear radio-frequency trap and laser-cooled to form a line, separated by about 5 μm. All of the ions are initially prepared in a standard state and a given algorithm is executed by applying a series of 50–100 laser pulses on particular individual or pairs of ions along the chain. Monroe told physicsworld.com that this “involves a few software steps: first we compile the algorithm from ‘textbook’ operations into the operations that are native to our system”.
Primitive operations
These native operations are then further broken down into pre-calculated laser pulse shapes, which depend upon the position of the particular ions in the chain. “Each of these primitive operations has 99% accuracy or so, and indeed when we concatenate 50–100 of these operations, the net accuracy of the algorithm is in the 70–90% range, depending on the details,” he adds. The team was able to implement these gates with greater than 98% fidelity.
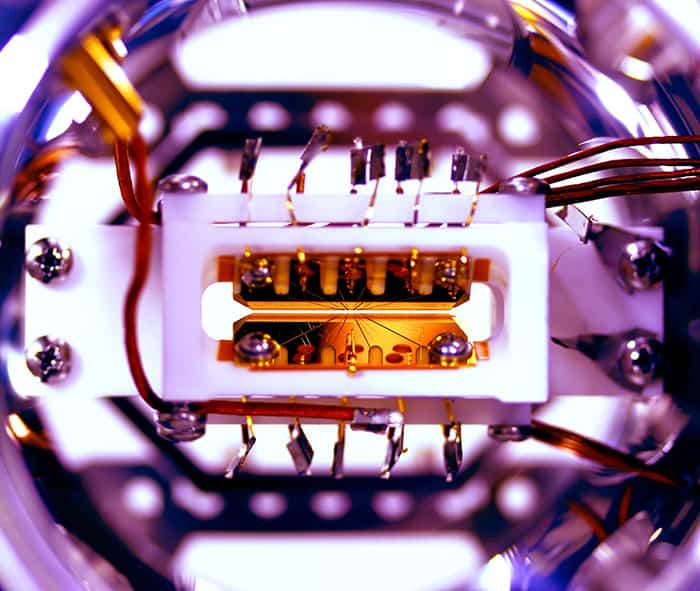
The researchers implemented some quantum algorithms, to see how the system fared as their five qubits worked in tandem. They ran two simple quantum algorithms – the Deutsch-Jozsa and Bernstein-Vazirani algorithms – that perform mathematical functions in a single step. While these can easily be performed on a normal computer, it would take several operations. They also ran a quantum Fourier transformation (QFT), which is a fundamental step in other more complex algorithms. “The QFT involves two-qubit gates between all possible pairs of qubits, and it has never been demonstrated,” says Monroe. Debnath says that it is “a very useful protocol used in the Shor’s factorization and a variety of other phase-estimation problems”, adding that these algorithms “show the flexibility of the processor as a programmable device”.
Atomic perfection
According to Monroe, trapped-ion qubits are more flexible as compared with their solid-state counterparts – they can be replicated and scaled up with negligible variation from qubit to qubit, and can be externally reconfigured using lasers and their quantum states can be initialized and read out with near 100% accuracy. Their downside though, is that they perform a quantum operation relatively slowly (gate times of microseconds or longer). And, as with most quantum systems, the usual challenge “is making sure that the ‘quantumness’ is well preserved as the system is scaled to more qubits”, says Debnath.
More problematic though is the fact that the practical engineering of trapped-ion qubits lags behind that of silicon and solid-state platforms because there are very few industrial companies that make these ionic devices, according to Monroe. “Sandia National Laboratories is one of the few places that manufacture monolithic chips with microfabricated electrodes for the reliable loading and trapping of atomic ions, and the future of this technology will depend upon Sandia and other places like Honeywell, Inc. to produce more varieties of these chips”, says Monroe.
He adds that his Maryland group has been aided by the fact that it uses a particular atomic species of ytterbium (171Yb+) that is also used for atomic clocks, and the control laser they use is industrially produced and has been reliably engineered. “The ion-trap community is dominated by university and federal research laboratories, with students and postdocs instead of professional systems-engineering staff. It can take many years to obtain the infrastructure to get these devices to work reliably and repeatedly.” Despite these issues, Monroe, Debnath and the team are convinced that ion-trap computing has the potential to be scaled up – both by increasing the number of qubits as well as by increasing connectivity between qubits by using photons – to a fully fledged quantum computer.
The work is published in Nature.