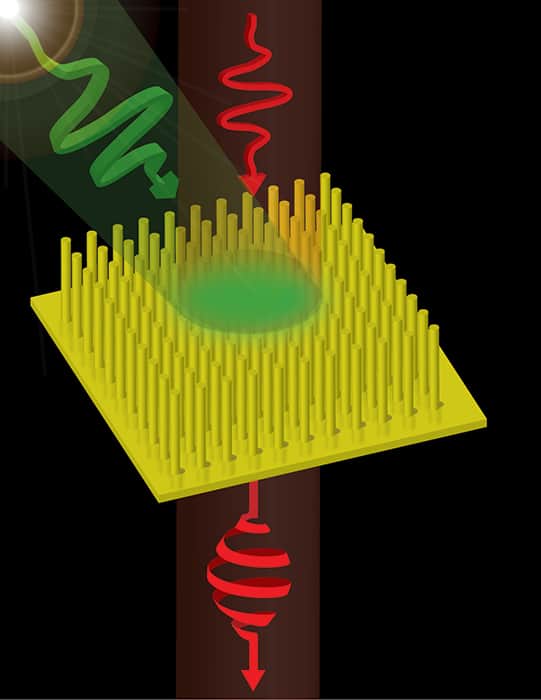
A new and quicker way of modulating the polarization of light pulses has been unveiled by researchers in the UK. The technique uses a second laser pulse to control polarization and could be used in a range of practical applications including pharmaceutical development.
Devices that modulate the polarization of an optical signal play important roles in a range of technologies. The data capacity of an optical fibre can be increased, for example, by encoding information into different polarization states of light that are then transmitted simultaneously. Today, the speed that this multiplexing can be achieved is restricted by how quickly the polarization can be modulated.
Most active modulators available today are hybrid optical and electronic devices, which means that they are inherently slow. Some ultrathin optical metasurfaces can significantly alter polarization, but these have been passive devices that are not suitable for making an active polarization modulator.
Gold nanorods
In the new research, Luke Nicholls and colleagues at King’s College London used a metasurface comprising a grid of 400 nm-long gold nanorods in an aluminium-oxide matrix. This structure supports both an ordinary light wave, in which the electric field oscillates perpendicular to the axes of the nanorods, and an extraordinary wave, in which it oscillates along the nanorods. The material’s refractive indices for these two waves can be very different.
For the ordinary wave, the material’s refractive index remains almost unaffected by frequency. For the extraordinary wave, however, the refractive index changes not only its magnitude but even its sign. When the frequency of light drops below the natural oscillation frequency of the electrons in the gold nanorods (the effective plasma frequency), the refractive index becomes negative. This means that the metamaterial inverts the phase of the extraordinary wave: “You’re giving [the extraordinary wave] a phase delay relative to the ordinary wave,” says Nicholls, “which in turn flips the polarization state.”
It would be impracticable in telecommunications, and impossible in many sensing applications, to constantly change the signal’s frequency to achieve a different polarization. However, the team had another trick up its sleeves. “When you shine an intense laser pulse on the material, it’s absorbed by the electron gas of the material,” explains Nicholls, “and luckily for us the refractive index is closely linked with the energy of the electrons.”
Frequency shift
The effective plasma frequency can therefore be shifted to lower frequencies with a brief “control pulse” immediately before the signal pulse. The signal frequency, therefore, can be below the effective plasma frequency – meaning its polarization is flipped – under normal circumstances, but above the effective plasma frequency – meaning its polarization is unaffected – if the metamaterial has just been hit by the control pulse. The team also showed that one pulse can perform both control and signal functions: the leading edge of the pulse controls how the polarization of the rest of the pulse is affected, with a more intense pulse being rotated more strongly.
“More generally there is a resonance in one component of the transmitted field (the extraordinary wave) and no resonance for the perpendicular component (ordinary wave),” explains Nicholls. “Like any resonance there is a phase shift between frequencies below and above the resonance. The output polarization of a signal beam at a set wavelength is thus controlled by changing the relative position of the resonance to the signal pulse with the control pulse and inducing a phase shift in the extraordinary component of the transmitted signal pulse.”
Relaxation limit
The researchers modulated wave polarization at frequencies up to 300 GHz – the fastest achievable with current technology is 40 GHz. It may be possible to go even faster: “At the moment, our speed is limited by the relaxation of the electrons back to the ground state,” says Nicholls, “We are looking at ways of understanding this process more and speeding it up.”
In principle, rotating the polarization of light faster could increase the number of signals squeezed into a single optical fibre. However, the researchers believe the technique could find other uses. It is often crucial that pharmaceutical molecules are in left-handed forms because right-handed forms can be ineffective or even toxic. The proportion of molecules in left- and right-handed forms can be measured by analysing how a solution affects different polarizations of light, a technique called polarimetry.
“If they can switch the polarization on a timescale on which the chemical reactions are happening,” says Nicholls, “then hopefully drug developers can understand what processes are leading to these bad orientations and potentially cut them out.”
Significant improvement
Andrea Alù of The University of Texas at Austin, who was not involved in the research, describes it as “a significant improvement” although “not necessarily surprising”: he notes that a recent paper from US researchers in the same journal describes similar effects with reflected waves. Nicholls says that this paper was submitted after that of the King’s group’s paper.
Alù points out that the effective plasma frequency resonance is a maximum in energy dissipation and therefore signal loss. This could make the process untenable in telecommunications, but he believes the set-up is promising elsewhere: “If you want to do ultrafast chemical sensing that’s not possible at present,” he says, “loss is not necessarily the first metric you look at.”
The research is described in Nature Photonics.