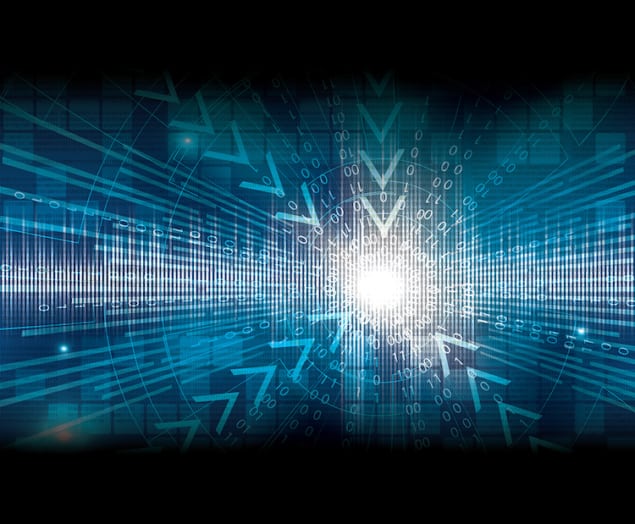
A new low-power technique for detecting the spin of electrons in a non-magnetic system could aid the development of spintronics devices that work using ferroelectricity rather than ferromagnetism. Such devices may eventually form the backbone of a new generation of efficient, low-energy computer processors, and thus help maintain progress in high-speed information processing.
For more than half a century, computing power has increased exponentially. Recently, however, this “Moore’s law” growth – named for Intel co-founder Gordon Moore, who predicted in 1965 that the number of transistors per square inch on integrated circuits would double every year for at least a decade – has slowed, as it is becoming increasingly difficult to make conventional transistors smaller than they already are. Researchers are therefore seeking ways of allowing computing power to continue to grow, even as traditional sized-based scaling runs up against fundamental limits.
Among the many solutions being investigated are those that seek to reduce power consumption in the field-effect transistors (FETs) that form the basis of modern silicon computer chips. One way to do this would be to replace conventional transistors with an alternative version that does not require a continuous power supply to maintain its ON or OFF state.
Spintronics circuits are smaller and more efficient
Spintronics technologies, which use electrons’ quantum spin (or intrinsic angular momentum) rather than their charge to store and process information, may offer a way to reach this goal. Since electronic spins can point “up” or “down”, this binary property can be used to perform logical operations in spintronic circuits in much the same way as electric charge is used in electronic circuits. The key advantage is that when an electron’s spin switches direction, its new state is stored permanently (that is, it is “non-volatile”). Hence, spintronics circuits do not require any additional input power for their states to remain stable.
Spintronic circuits do, however, have a major drawback for efficiency advocates. In spintronics, information is carried or manipulated via spin currents, which consists of electrons with opposite spins moving in opposite directions. These currents are usually generated using ferromagnetic materials. That’s a problem, because the magnetization of such materials cannot be switched except by applying very strong magnetic fields or currents. Hence, in any practical, switchable device, the energy advantage associated with non-volatile storage would quickly be wiped out.
A new way to control spins
Researchers at the Spintec Laboratory (CNRS/CEA/Université Grenoble Alpes) and the CNRS/Thales Laboratory led by Jean-Philippe Attané and Manuel Bibes have now developed a new, lower-power way to control spin currents. Their method uses the ultrathin layer of conducting electrons – known technically as a two-dimensional (2D) electron gas – that develops at the interface between strontium titanate (an electrical insulator in its pure state) and a covering layer of aluminium.
The researchers began by injecting a spin current from a ferromagnetic nickel-iron alloy into the strontium titanate (SrTiO3). Once the electrons are confined in the 2D electron gas, their spin couples to their momentum thanks to a phenomenon known as spin-orbit interaction. “This effect converts the spin current into conventional charge current, which allows us to detect the injected spins,” Attané and Bibes explain.
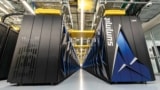
A cool spin on supercomputers
SrTiO3 behaves like a ferroelectric material
Next, the team applied a voltage across the insulating SrTiO3 beneath the electron gas to tune the spin-orbit coupling, and thus the direction of the charge current. At this point, they discovered that the insulating SrTiO3 was behaving like a ferroelectric material – that is, it had a permanent electric dipole moment, just as a ferromagnetic material has a permanent magnetic dipole moment. This is a significant benefit, since electric dipole moments can be oriented using electric fields, which are much easier to control than the magnetic fields required to switch the magnetization of ferromagnetic materials.
Attané and Bibes and their colleagues also confirmed that the direction of the SrTiO3’s overall electrical polarization depends on the polarity of the applied voltage. This electrical polarization remains even when the electric field is switched off, meaning that they could permanently control the spin-orbit coupling and thus the direction of the charge current produced from the spin current.
The research is detailed in Nature.