Liquid-crystal displays that let viewers watch images in 3D are the latest success story in the electronics industry – the only problem being that special spectacles are required. But, as Jonathan Mather explains, liquid-crystal technology is also ideal for "glasses-free" 3D displays
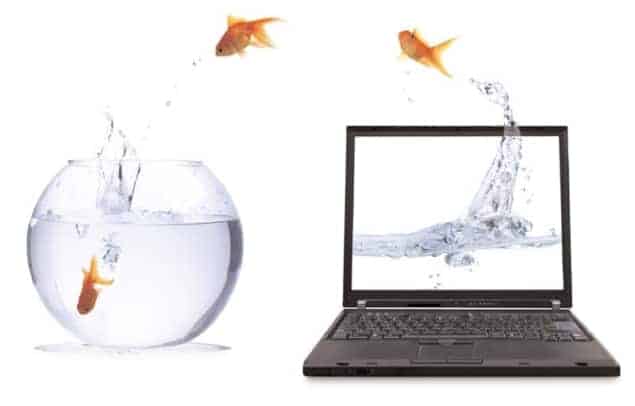
“Wow, that’s amazing” was at least one visitor’s reaction to last year’s Summer Science Exhibition at the Royal Society in London. Their enthusiasm was not primarily due to the presence of the Queen, who had earlier opened the exhibition as part of the society’s 350th anniversary celebrations. Rather, their excitement was down to the latest liquid-crystal displays on show from Sharp Laboratories in Oxford. Viewers were able to watch moving images in 3D on a laptop – without the need for special glasses. They could see pigeons being fed in St Marks Square, Venice, with the birds apparently descending from their shoulders, or they could indulge in a 3D adventure as they pursued the bad guys in the interactive computer game Quake.
The glasses-free 3D display on show at the Royal Society was just the latest example of the revolution wrought by liquid-crystal displays, which now allow moving images to be viewed on everything from mobile phones and 46-inch flat-screen televisions to hand-held personal electronic games and the iPad and other tablet devices. Yet it is amazing to think that it is only 40 years since the key patent was filed that marked the birth of the modern liquid-crystal display – a technology so successful that its acronym, LCD, is instantly recognized even by non-scientists. Although organic light-emitting diodes (OLEDs), plasmas and “electronic ink” are also changing the nature of the modern display, it is the remarkable properties of liquid crystals that are now at the forefront of 3D display technology.
Imaging in 3D
Watching images in 3D without glasses is a truly astounding experience, but let’s first look at how this fits in with other 3D imaging techniques. There are three main techniques – stereoscopic, holographic and volumetric – all of which operate on the same principles regardless of whether the screen uses liquid crystals, plasmas or OLEDs. They each have advantages and disadvantages in terms of realism, complexity, size and cost, but the most commercially viable method, which is used in the bulk of the 3D televisions taking the high street by storm, involves showing a different perspective of an image to each of our eyes. This “stereoscopic” technique mimics the real world, where each eye sees a different perspective and the brain “fuses” the two images together to create a 3D perception of the surroundings (figure 1a).
The task of separately displaying images to the left and right eye has been tackled in a variety of ingenious ways over the years. Trialled at cinemas as far back as the 1950s, the approach that many people will be familiar with involves the user wearing glasses with separate red and blue coloured lenses on the left and right eye, respectively. The idea here is that an image is split into red, green and blue channels, with the left eye seeing only the red image and the right eye seeing only the green and blue images.
More recent systems do away with coloured lenses and instead use glasses that alternately transmit and block light to each eye. In other words, the lenses act as “optical shutters” so that at any one moment one eye can see a still image, but the other cannot. If we label the successive still images of a movie L1, R1, L2, R2, L3, R3 and so on, then the left eye sees only the “L scenes” and the right eye sees only the “R scenes”. These glasses require various bit of electronics to make them work, while the scenes themselves are updated at frequencies of typically 120 Hz or 240 Hz. (An alternative approach – common with projection screens found in pubs to watch sport on – is for the L and R scenes to be displayed with different polarizations, which requires the user to wear dark glasses containing lenses with different polarizations.)
The images produced using this stereoscopic approach can jump out of the screen with surprising realism. However, stereoscopic images are not perfect because all objects in them are in focus, regardless of their intended 3D position. In the real world, in contrast, different depths of a 3D image are in focus at different positions. One technique for creating 3D images that does deal with focus correctly is holography (figure 1b). Holograms are created by recording in a photosensitive material the interference pattern created when coherent reflected light from an object overlaps with a coherent reference beam of the same wavelength. The pattern is stored as a change in absorption, refractive index or thickness of the photosensitive material and a copy of the object can be recreated by illuminating the pattern with a read-out laser. A 3D hologram is essentially like having a stack of high-resolution 2D pictures, where each picture represents a different image plane.
The big advantage with a 3D hologram is that a viewer’s perception of three-dimensionality is total because to change from looking at an object near the front of the scene to an object at the back, the viewer needs to adjust their eyes’ focus. Unfortunately, creating and controlling optical wavefronts with sufficient precision to generate realistic holographic images requires displays with pixel densities typically thousands of times higher than are found in today’s commercial LCDs, as well as prodigious amounts of computer processing power to handle the volumes of data needed. So although their images are superior, further technical innovation is still required before holographic displays become a commercial reality.
Stereoscopy, in contrast, relies on the fact that our brains are good at deducing depth from our right and left eyes having different perspectives of an image. In practice this means that a stereoscopic display can create a 3D image using only twice the amount of data that a “normal” display needs to make a 2D image, which is why they are proving so commercially promising.
The third approach to making 3D displays is to do away with conventional 2D pixels arranged in a plane and instead use 3D, volumetric pixels, or “voxels”. One way of creating such voxels is to use projectors shining at a spinning screen (figure 1c). By synchronizing the projectors with the screen, light can be reflected off the screen at any position within the cylindrical volume that it sweeps out. Although volumetric displays can create a strong 3D impression, one snag is that the light projected into the volume of the display is free to propagate throughout this space. This can make items transparent, with objects supposedly hidden behind others tending to “shimmer through” those in front. Volumetric displays also tend to be fairly bulky.
Glasses-free stereoscopy
So far we have described glasses-based stereoscopic 3D displays, but what everyone wants is to do away with the glasses altogether. This is an active area of research being pursued by probably every major displays company and from which new consumer products are now starting to emerge. Nintendo, for example, has already released its glasses-free Nintendo 3DS games console, while 3D mobile phones are available from Sharp.
All such glasses-free displays are based on stereoscopy and the challenge is to ensure that different images are directed to each eye. There are three main methods of achieving this, each of which has its own advantages and disadvantages depending on what it might be used for. The most common approach is where the user has to sit in a fixed position in front of the screen, and this is used, for example, on the Nintendo 3DS, Sharp’s LYNX 3D SH-03C mobile phone and in the display on the back of Fujifilm’s W3 3D camera. The next approach involves the display tracking the viewing position of the user, and although there are currently no products using this currently on the market, prototype designs have been shown at industry exhibitions in recent years. The final tack is “multi view”, which is already found in some glasses-free 3D televisions, although they have not made big inroads into the market as yet partly because it is not easy to generate multi-view 3D without changing broadcasting standards.
The “fixed-position” method assumes that the user views the display head-on so their gaze is at 90° to the display itself (figure 1a) – an assumption that is valid for most mobile devices. The image is separated into tiny stripes L, R, L, R, L, R, with all the L images being sent to the left eye and all the R images being sent to the right eye by means of a physical device known as a “parallax barrier” (figure 2). This technique, which has been known for almost 70 years, could of course be applied to any images – be they photos or paintings – not just an LCD display, provided of course that the left and right images can be interlaced into left and right image stripes to work with the parallax barrier.
A disadvantage of the parallax barrier is that because each eye is allowed to see only half the pixels, light travelling in the “wrong” direction – i.e. from an L stripe to the right eye or from the R stripe to the left eye – is absorbed by the barrier. This cuts the intensity from the display by about half and reduces the resolution. In practical terms, this means that when the display is being used in conventional 2D mode, the parallax barrier should be removed. In most 3D displays, such as Sharp’s 3D mobile phone, this is achieved by making the barrier from a liquid-crystal layer that can be turned on or off electrically.
It would of course be much more efficient to dispense with a parallax barrier and instead use lenses, which are transparent, to redirect the L and R light to the appropriate eye. Indeed, researchers have already developed high-quality cylindrical lenses using liquid crystals that can do just that. The principle is simple: as the refractive index of liquid crystals varies with voltage, lenses made from these materials can be turned “on” when a voltage is applied and “off” when the voltage is removed. These cylindrical liquid-crystal lenses take the place of the parallax barrier, redirecting the light in the correct direction (figure 3). This technology is likely to double the efficiency of glasses-free 3D displays in the future, with many companies known to be actively carrying out research into them.
One drawback of parallax technology is that the user has to sit in a certain position relative to the screen. The “tracked viewing position” technique, in contrast, allows 3D screens to be viewed without glasses from any angle by tracking the user’s head position. This could be achieved by, for example, fitting a laptop with a forward-facing web camera to identify the location of the user’s face and eyes. Indeed, this technology is already common in many digital cameras sold on the high street to ensure that a face automatically becomes the centre of focus. All that is then needed for glasses-free 3D viewing is an automatically adjustable parallax barrier that can change the angle at which the left and right images are seen. The camera can then identify the position of the user, while the parallax barrier directs the left and right perspectives at the appropriate angle.
This adjustment can be carried out using face-tracking algorithms written onto image-processing chips, which operate very efficiently, meaning that not too much processing power is required. The camera can also monitor how far a user is sitting from the screen and adjust the images accordingly. In practice, the viewer can move up to 30 cm from the ideal viewing distance, while their side-to-side movement is limited to about ±30° from the normal. Accommodating more than one user is in principle possible, but the complexity of the system is significantly increased. In addition to the viewer being free to move around, the other advantage of the tracked-viewing-position system is that if the image happens to be from a computer-generated scene, the viewpoint could be adjusted according to the user’s position. For example, a viewer could literally look around the side of an object (a cube say) to bring previously unseen faces of it into view.
As for the third method for generating glasses-free 3D – multi-view – its goal is to work with a wide range of viewing positions and multiple viewers. To do this the display outputs not just two perspectives but typically eight or more. The user can then position their eyes to see perspectives 1 and 3, or 2 and 4, etc, so the 3D effect can be achieved from a wide range of angles. A multi-view system with, say, eight views requires eight times greater resolution than a 2D system, and some ingenuity is required to synthesize the eight views or transmit them in the available television bandwidth. Nevertheless, this technology is probably the strongest contender for glasses-free 3D television, with Philips and Toshiba both having already launched a multi-view television onto the market.
Fast forward
As we have discussed, Sharp has already designed and built a liquid-crystal screen on one of its mobile phones that functions as a switchable parallax barrier. Used in tandem with a conventional liquid-crystal display provided with stereoscopic input data, this system gives high-quality glasses-free 3D images. However, the electronic media industry has a vision of the future in which 3D displays are not just a niche product but an integral part of modern life. That means home-cinema systems showing 3D movies, computer games being played with an immersive 3D environment, and holiday photographs being presented with depth.
We can therefore expect a variety of 3D generation methods to become available for the different applications, and within each method we can expect improved optical technologies, and new related technologies such as those enabling 3D interaction. It is highly probable that all such devices will exploit the particular electro-optical properties of liquid crystals. The products on display at the Royal Society exhibition last summer, which are already coming on the market this year, are just the start.