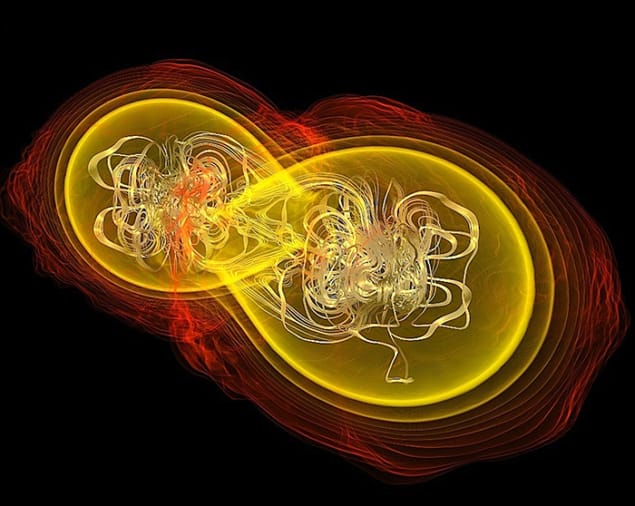
New insights into the properties of neutron stars have come from two independent analyses of gravitational waves from the GW170817 neutron-star merger. The work was done by teams led by Farrukh Fattoyev at Indiana University Bloomington and Eemeli Annala at the University of Helsinki. The teams used different methods to calculate the relationship between the radius and mass of neutron stars and came up with the same result.
In October 2017 the LIGO and Virgo detectors made the first-ever observation of gravitational waves from two neutron stars as they spiralled into each other and then merged to form a black hole. The observation is also notable as the first time that electromagnetic radiation was detected from a gravitational-wave event.
GW170817 offers astrophysicists new and exciting information about the equation of state (EOS) of neutron stars. The EOS describes the complex behaviour of the dense nuclear matter that makes up neutron stars. Little is currently known about the EOS because it is difficult to glean information from conventional astronomical observations and because theoretical calculations of dense nuclear matter are extremely difficult to do. Before GW170817, the most important piece of information about the EOS came from observations published in 2010 and 2013, which showed that neutron stars can have masses at least as large as two solar masses.
Tidal distortions
Just before the GW170817 neutron stars merged, the shapes of both stars are distorted by their mutual gravitational attraction – with the nature of the distortion being defined by the EOS. This tidal distortion affects the gravitational waves emitted by the pair, which means that careful analysis of LIGO-Virgo data should provide valuable information about the EOS.
LIGO Scientific Collaboration member Francesco Pannarale from Cardiff University explains: “The EOS determines the radius of a neutron star, and how easily it can be distorted as it experiences the tidal forces generated of another object, including another neutron star”. “This distortion is quantified in the tidal deformability, which was constrained by the analysis of GW170817.”
Fattoyev’s team used this constraint to assess experimental data from nuclear physics experiments that measure properties of protons and neutrons in atomic nuclei. “The researchers used the constraint on tidal deformability to determine a constraint on the neutron skin thickness – the difference between the radius of a neutron and a proton,” Pannarale says. “They then contrast the prediction to experimental skin thickness measurements. Right now the two values are compatible, but future experiments will provide a more accurate measurement of the neutron skin thickness.”
Thicker or thinner skins
Indeed, future experiments could lead to new discoveries about the elusive properties of matter at extremely high densities. “If the skin thickness value measured on Earth became no longer compatible with the value derived from gravitational-wave observations, it would mean that matter suddenly behaves differently from some density onward,” Pannarale explains. “Matter would undergo a phase transition, indicating that the way in which terrestrial and astronomical results are connected must break down.”
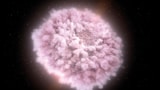
A new cosmic messenger
Taking a different approach, Annala’s team used the tidal deformability value to narrow the range of possible EOS models of neutron stars. “The researchers tried many possible EOS models where the GW170817 constraint on tidal deformability is obeyed, two-solar-mass neutron stars are allowed, and theoretical results at low and high density are respected,” says Pannarale. “Once they narrowed down the possibilities, they ended up with around 100,000 EOSs, allowing them to make predictions about neutron star properties.”
Using their results, both groups separately calculated a relationship between the mass and radius of neutron stars. “Both teams determined that the maximum radius of a 1.4 solar-mass neutron star is 13.6 km,” Pannarale explains. “While the two values are in very good agreement, they stem from very different approaches, indicating that the conclusions of the two teams are solid.” The remarkable result is promising for future studies of neutron star properties, and could soon be improved further through forthcoming observations of merging neutron stars.
Papers by Annala’s team and Fattoyev’s team appear in Physical Review Letters.